Table of Contents[Hide][Show]
Many of the health benefits attributed to fruits, vegetables, nuts, legumes, and whole grains are due to the way the fiber in these foods impact the gut microbiome.
Fiber is the quintessential example of a nutrient that isn’t labelled as essential but that is absolutely fundamental for our health. What separates fiber from other carbohydrates is that the ways the constituent saccharides link together are not compatible with our digestive enzymes; our bodies just aren’t capable of breaking apart those types of molecular bonds. Instead, fiber passes through the digestive tract mainly intact. And once it reaches the colon, the magic begins: fiber serves as a substrate (i.e., food) for the wide range of bacteria and other microbes living in our digestive tract, collectively referred to as our gut microbiomes!
Fiber Feeds the Gut Microbiome
The term gut microbiome refers to the massive collection of bacteria and other microbes that inhabit our gastrointestinal tract, and their metabolic byproducts. And “massive” is far from hyperbole: An estimated 30-100 trillion bacteria comprise the microbiota, collectively weighing around 4.5 pounds! These bacteria include a mixture of commensal (neutrally existing), probiotic (mutually beneficial, also called symbiotic), and pathogenic (harmful to us) organisms, and can consist of any of 35,000 species known to inhabit the human gut. Every person’s gut contains approximately 400 to 1,500 different bacterial species of the possible 35,000 that are well adapted to survive in the human gastrointestinal tract, although thirty to forty species of bacteria will dominate an individual’s gut microbiota, accounting for about 99% of the microorganisms present in our gut.
We have a symbiotic relationship with our gut microbes—we are the host and they are symbionts. In exchange for food and shelter, they contribute to our wellbeing via metabolic, trophic (digestion and nutrient absorption) and immunologic functions that exceed our own physiological capabilities. In fact, the gut microbiome can be considered a virtual organ, owing to the fact that our health is dependent on its activities.
Our gut microbes perform many different essential functions that help us to stay healthy. These include digestion, vitamin production, detoxification, regulation of cholesterol metabolism, providing resistance to pathogens, immune regulation, neurotransmitter regulation, regulation of gene expression, and more! In fact, every human cell is impacted by the activities of our gut microbes. A healthy gut microbial community is essential for our health. And, the converse is also true: An aberrant gut microbiome has been linked to conditions as wide-ranging as cancer, obesity, diabetes, cardiovascular disease, anxiety, depression, neurodegenerative diseases, autism, autoimmune disease, ulcers, IBD, liver disease, gout, PCOS, osteoporosis, systemic infections, allergies, asthma, and more! A healthy diversity of the right kinds of microorganisms in the gut is one of the most fundamental aspects of good health.
The composition and metabolic activity of the community of microbes in our guts is influenced greatly by the foods we eat, most notably the amount and types of fiber.
The THREE Best Probiotics
Bacillus/Spore-based
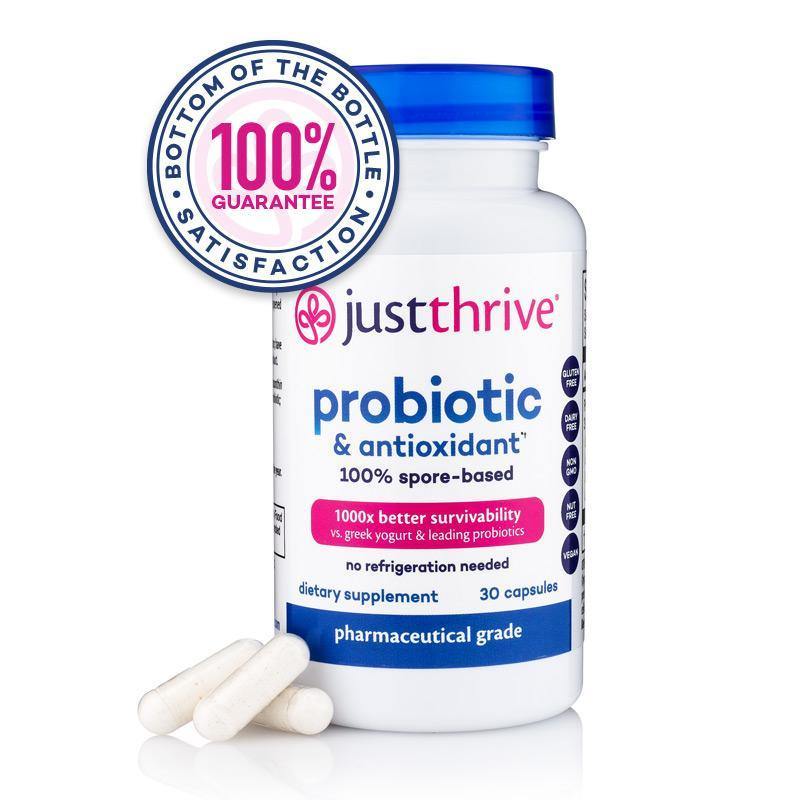
Best for a general probiotic, if you were recently on antibiotics, or for digestion issues (especially abdominal pain, bloating, flatulence, diarrhea, and gas after meals).
Lactobacillus & Bifidobacterium
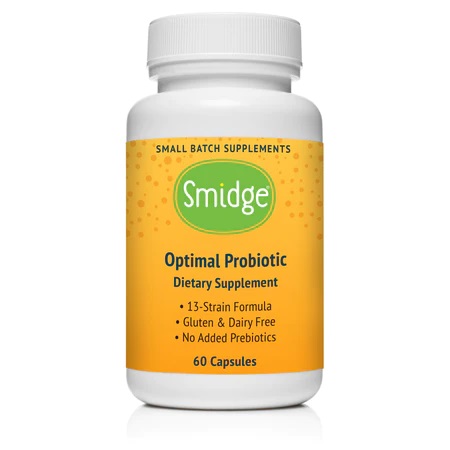
Best for if you don’t eat fermented foods, if you were recently on antibiotics, or if you have issues with constipation or gas.
Saccharomyces boulardii
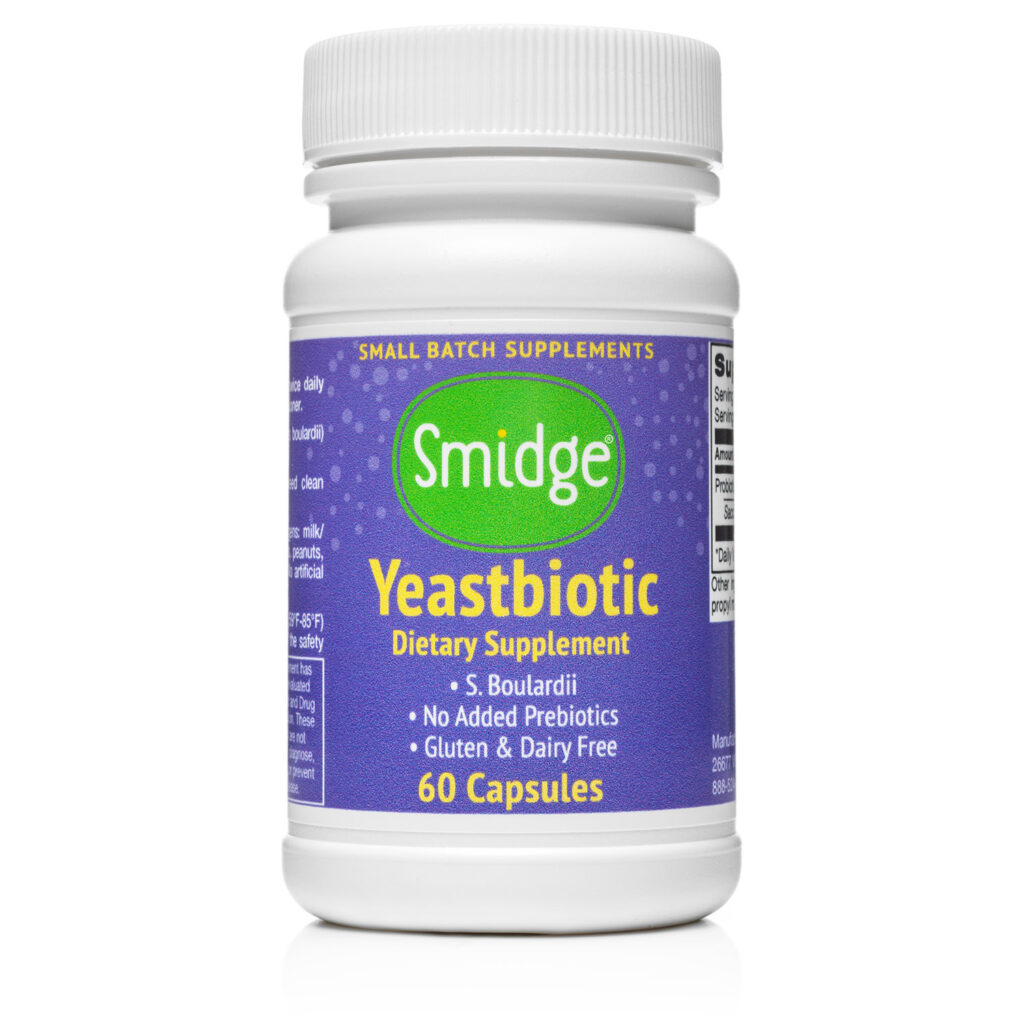
Best for if you have Candida issues, were recently on antibiotics, or have diarrhea.
Health Benefits of Fiber
Diets rich in fiber reduce the risk of many cancers (especially colorectal cancer, but also liver, pancreas, and others) and cardiovascular disease, as well as lower inflammation overall. Prospective studies have confirmed that the higher our intake of fiber, the lower our inflammation (as measured by C-reactive protein). In fact, a recent study showed that the only dietary factor that correlated with incidence of ischemic cardiovascular disease is low fiber intake (not saturated fat!); the more fiber we eat, the lower our risk. If someone has kidney disease, a high-fiber diet reduces their risk of mortality. If someone has diabetes, a high-fiber diet reduces their risk of mortality. A high fiber intake can even reduce the chances of dying from an infection.
Many of the health benefits attributed to fruits, vegetables, nuts, legumes, and whole grains are due to the way the fiber in these foods impact the gut microbiota. However, fiber has other effects, too, like regulating peristalsis of the intestines (the rhythmic motion of muscles around the intestines that pushes food through the digestive tract), stimulating the release of the suppression of the hunger hormone ghrelin (so we feel more full), and slowing the absorption of simple sugars into the bloodstream to regulate blood sugar levels and avoid the excess production of insulin. Fiber also binds to various substances in the digestive tract (like hormones, bile salts, cholesterol, and toxins) and, depending on the type of fiber, can facilitate either elimination or reabsorption (for the purpose of recycling, which is an important normal function for many substances like bile salts and cholesterol), both of which can be extremely beneficial—if not essential—for human health.
Want to know the top 25 foods for this awesome nutrient?
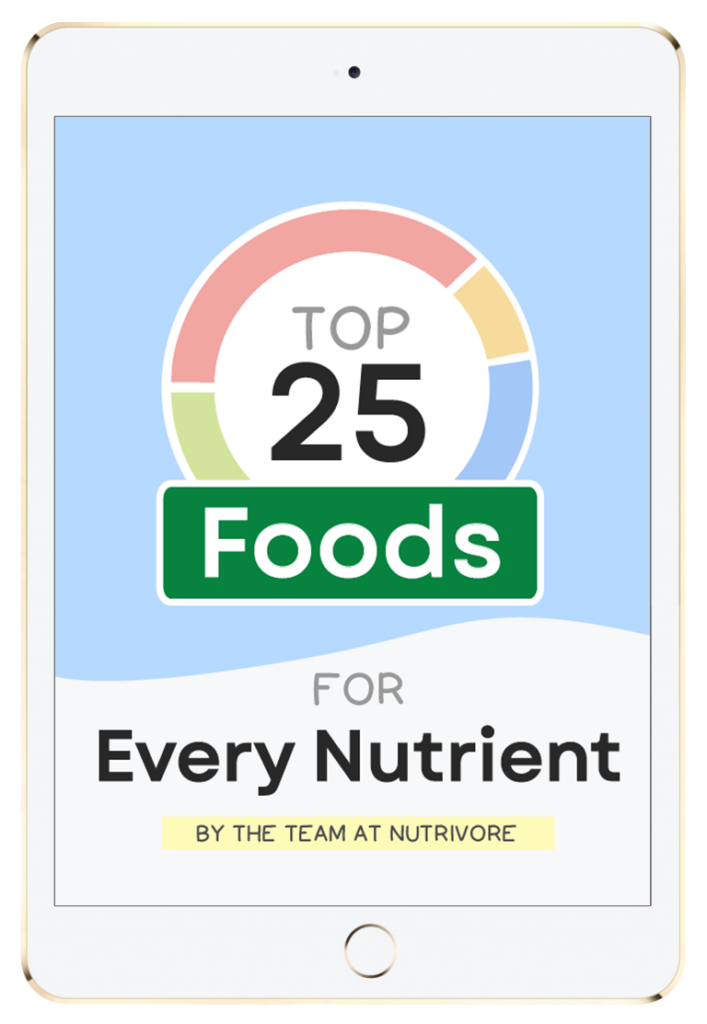
The Top 25 Foods for Every Nutrient
The Top 25 Foods for Every Nutrient e-book is a well-organized, easy-to-use, grocery store-friendly guide to help you choose foods that fit your needs of 43 important nutrients while creating a balanced nutrient-dense diet.
Get two “Top 25” food lists for each nutrient, plus you’ll find RDA charts for everyone, informative visuals, fun facts, serving sizes and the 58 foods that are Nutrient Super Stars!
Buy now for instant digital access.
Problems From Low-Fiber Diets
Given how important fiber is for supporting the gut microbiome, it’s no surprise that low-fiber diets have been associated with a wide range of health problems, including heart disease, diabetes, colorectal cancer, and a variety of gastrointestinal illnesses (such as constipation, IBS, ulcerative colitis, and hemorrhoids). Scientists have been exploring the mechanisms behind how low-fiber diets exert their effects on disease risk via the gut, helping us understand exactly what happens when our microflora get deprived of this important food source!
Even for conditions where low-fiber diets are sometimes advised, such as Crohn’s disease, the wisdom of reducing fiber isn’t always backed up by science. In one study of 1619 patients with Crohn’s disease or ulcerative colitis, people who avoided high-fiber foods had a significantly higher risk of disease flares than people who didn’t reduce their fiber intake. Likewise, a randomized, controlled trial involving Crohn’s disease patients found that the group consuming a high-fiber diet reported significantly better health-related quality of life and gastrointestinal function than the lower-fiber control group.
Everything You Need to Jump into Nutrivore TODAY!
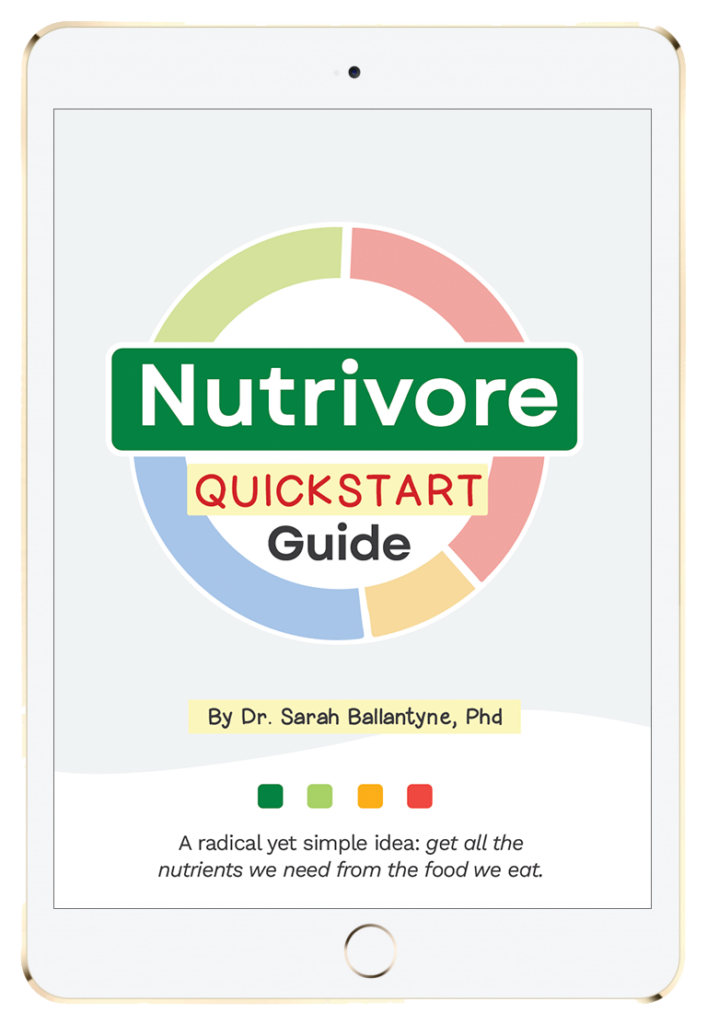
Nutrivore Quickstart Guide
The Nutrivore Quickstart Guide e-book explains why and how to eat a Nutrivore diet, introduces the Nutrivore Score, gives a comprehensive tour of the full range of essential and important nutrients!
Plus, you’ll find the Top 100 Nutrivore Score Foods, analysis of food groups, practical tips to increase the nutrient density of your diet, and look-up tables for the Nutrivore Score of over 700 foods.
Buy now for instant digital access.
Types of Fiber
Most of our dietary fiber intake comes from the cell walls of plants, where it acts like a skeleton and helps to maintain the plants’ shape and structure. However, special forms of fiber are also found in edible insects and fungi. There are many, many different types of fiber consisting of different length of carbohydrate strings composed of different saccharides, some with branches and some without.
Fiber can be broadly categorized as either soluble or insoluble:
Soluble fiber forms a gel-like material in the gut and tends to slow the movement of material through the digestive system. Soluble fiber is typically readily fermented by the bacteria in the colon, producing gases and physiologically active by-products (like short-chain fatty acids and vitamins).
Insoluble fiber tends to speed up the movement of material through the digestive system. Fermentable insoluble fibers also produce gases and physiologically active by-products (like short-chain fatty acids and vitamins). Insoluble fibers, such as cellulose and hemicellulose, are typically fermented more slowly by gut bacteria, and consequently move through the colon and add bulk to the stool (which is beneficial for regulating bowel movements and managing constipation).
Depending on the food in question, some foods have more insoluble fiber types and some have more soluble. While soluble fibers have the reputation of being the fermentable fibers, all types of fiber are fermentable at least to some extent by our gut microbiota.
Fiber can be more sophisticatedly classified based on the molecular structure. The major classes of fiber are as follows:
Cellulose
Cellulose is the main component of plant cell walls. Celluloses are identical to starch in the sense that they are long straight chains of glucose molecules (anywhere from several hundred to over ten thousand glucose molecules long), however the links between the glucose molecules are different than starch (they are in what is called a beta configuration), which make cellulose indigestible to humans. Celluloses are insoluble dietary fibers.
Our gut bacteria cannot ferment most cellulose particularly well (although cellulose is partially fermentable); however, cellulose is still very beneficial! One study showed that when combined with resistant starch, cellulose from wheat bran was able to shift resistant starch fermentation throughout a greater portion of the gut, nearly doubling the amount of resistant starch being fermented between the proximal colon and feces, and significantly increasing the concentration of butyrate in the distal colon. (Without the cellulose, the resistant starch was rapidly fermented in the caecum and proximal colon, leaving little left for further down in the colon.) A major benefit of this is the increased delivery of butyrate to regions of the colon where tumors commonly occur.
Cellulose is found in all plants, but foods that contain particularly large amounts of cellulose include bran, legumes, nuts, peas, root vegetables, celery, broccoli, peppers, cabbage and other substantial leafy greens like collards, and apple skins.
Hemicellulose
Hemicellulose is a common component of the cell walls of plants. In contrast to cellulose, hemicellulose is made of several types of sugar in addition to glucose, especially xylose but also mannose, galactose, rhamnose, and arabinose. Rather than forming long straight chains like cellulose, hemicellulose may have side chains and branches. Because of these variations, some hemicelluloses are soluble in water and some are insoluble, plus some forms are fermented by bacteria while others are not. Some non-digestible oligosaccharides (which are similar to resistant starch in that they resist digestion in the small intestine but can be degraded by bacteria in the colon) are classified as hemicellulose, such as the galacto-oligosaccharides present in legumes.
Hemicelluloses encompass a wide variety of fibers with a variety of roles in shaping the gut microbiota! Hemicellulose in the form of oligosaccharides appear to enhance levels of the extremely important probiotics Bifidobacterium and Lactobacillus. A systematic review and meta-analysis of 64 studies found that galacto-oligosaccharide consumption (along with fructan consumption) was associated with significantly higher levels of bifidobacteria and lactobacilli compared to other fiber types.
Hemicellulose in general is particularly high in bran, nuts, legumes, and whole grains, as well as many green and leafy vegetables.
Pectin
Pectin is soluble in water and highly fermentable (very little passes through to the colon, since it is so readily fermented by bacteria in the small intestine). Pectins are rich in galacturonic acid and can be found in several types of configurations (further subdividing this class of fibers by structure).
As with many fibers, the source of pectin (and subsequently, minor variations in its structure) matters in terms of usability by gut microbiota! For example, the extremely beneficial Faecalibacterium prausnitzii has been shown to grow on pectin derived from apples, but not pectin derived from citrus. In rats, a diet containing 6.5% citrus pectin enhanced the abundance of Bacteroides species, while a different study showed that a diet containing 7% apple pectin decreased the abundance of Bacteroides (while also decreasing levels of Alistipes and increasing levels of species belonging to Anaeroplasma, Anaerostipes, and Roseburia). The best way to benefit from pectin is to consume a wide range of food sources of it.
Pectins are found in all fruits and vegetables but are particularly rich in certain fruits, including apples and citrus fruits, and are also found in legumes and nuts.
Lignin
Lignin is a type of fiber with lots of branches made of chemicals called phenols (rather than sugar molecules). Phenols are currently being studied for a variety of health-related effects, including antioxidant actions (for example, it is the phenolic compounds in olive oil that appear to be responsible for its cardiovascular health benefits). Lignin is unusual because it lacks an overall defining structure. Instead, it consists of various types of substructures that appear to repeat in a haphazard manner. Lignins are insoluble and can be metabolized by the gut microbiota into various bioactive metabolites, such as enterolignan. One study used lignin-rich fractions from Brewer’s Spent Grain and found that, using an in vitro metabolic model of the colon, the microbiota were able to successfully metabolize the lignin into several phenolic compounds without inhibiting the growth of beneficial microbes.
Most commonly a component of wood, food sources include root vegetables, vegetable filaments (like the stems of leafy greens and the strings in celery), many green, leafy vegetables, wheat, and the edible seeds of fruit (such as berry seeds and kiwi seeds).
Chitin
Chitin is similar to cellulose in the sense that it is made of long chains of glucose (in the case of chitin, it’s actually long chains of a particular derivative of glucose called N-acetylglucosamine) and also has amino acids attached. Chitins are insoluble in water and are fermentable, albeit weakly.
In studies, chitin from mushrooms and insect exoskeletons has been shown to support the growth of important probiotic species from Bifidobacterium (including Bifidobacterium animalis), Lactobacillus, Akkermansia, and Bacteroides genera while also decreasing the abundance of the inflammatory microbe Desulfovibrio. In mice, chitin oligosaccharides (produced from chitin digestion) are also able to modulate the gut microbiota to combat diet-induced metabolic syndrome in mice, inhibiting the destruction of the gut barrier, restoring the Firmicutes to Bacteroidetes ratio to what it was before high-fat feeding.
Chitin is interesting because this fiber is found not only in plants and fungi but also in the exoskeletons of insects and in the shells of crustaceans.
Chitosan
Chitosan is similar to chitin in the sense that is composed of a long chain of N-acetylglucosamine molecules, but it also contains randomly distributed D-glucosamine molecules (like cellulose, linked in a beta configuration). Chitosan is a very unique fiber. It is soluble in acidic environments so it starts its journey through the digestive tract as a soluble fiber in the stomach, but when the acidity of the chyme (stomach contents) is neutralized in the small intestine (by pancreatic secretions), it becomes insoluble. It is also fermentable (much moreso than chitin).
In mice, chitosan increases gut microbial diversity (along with a general increase in Bacteroidetes and a decrease in Firmicutes) and decreases levels of potentially pathogenic genera Escherichia and Shigella. In diabetic mice, chitosan has also been shown to reshape the microbiota to induce an anti-diabetic effect, relieving dysbiosis by raising levels of the extremely important probiotic Akkermansia muciniphilia and suppressing the growth of Helicobacter.
Chitosans are naturally found in the cell walls of fungi but are also produced as a functional fiber by treating shrimp and other crustacean shells with sodium hydroxide.
Gums
Gums are a diverse group of fibers that plants secrete when they are damaged. They are very complex molecules that contain a variety of types of sugars as well as acids, proteins, and minerals. Gums are soluble and highly viscous fibers and are also fermentable. Isolated (functional fiber) versions are used in food manufacturing as thickening and gelling agents (like guar gum and xanthum gum). Some gums used in food manufacturing increase intestinal permeability through an action on the tight junctions between epithelial cells (one of those cases of the isolated concentrated compound being a problem but the small amount naturally occurring in whole foods being fine).
Various gums have demonstrated prebiotic properties in studies. In a study using human fecal samples, partially hydrolyzed guar gum was able to stimulate the growth of Parabacteroides, a bacterial genus inversely associated with IBS and ulcerative colitis. An in vivo human study also showed that partially hydrolyzed guar gum stimulated the growth of probiotic Bifidobacterium and other beneficial butyrate-producing bacterial strains.
β-glucans
β-glucans (more technically β(1,3)-glucans) are closely related to gums and are also soluble (a minority are insoluble), viscous and fermentable.
β-glucan feeds anaerobic microbes in the gut and can significantly increase levels of butyric and propionic acids. Grains rich in β-glucan, such as barley, have been shown to increase levels of extremely important probiotic Bifidobacterium, Lactobacillus, Dialister, and Roseburia species. In an extensive review of the health effects of β-glucan, researchers concluded that this fiber’s actions upon the gut microbiome, including enhancing the production of short-chain fatty acids, contributes to its anti-cancer, anti-inflammatory, anti-diabetic, and immune-modulating effects.
β-glucans are found in some grains (mainly oats and barley, but also rye and wheat), fungi (yeast and mushrooms, particularly those mushrooms that are used medicinally like shiitake and maitake), and some types of seaweed (mainly algae). β-glucans are the fiber in oats that are largely responsible for their unique health benefits among grains.
Mucilages
Mucilages are rich in the simple sugars xylose, arabinose, and rhamnose and have very complex structures. They are soluble and very viscous fibers, forming a thick gluey substance, and are produced by nearly all plants and some microorganisms. They can also be found in relatively large amounts in a variety of fruits and vegetables, including plantains, bananas, taro root, cassava, and berries. While soluble, mucilages are not particularly fermentable (only partially degraded by bacteria in our digestive tracts).
Mucilage has been shown to act as a prebiotic, enhancing the growth of the very important probiotic Lactobacillus, increasing short-chain fatty acid production, and reducing the population of harmful species of bacteria.
Mucilages are particularly concentrated in cacti and other succulents (like aloe), many types of seaweed (like agar agar algae), flax, chia and psyllium.
Fructans
Fructans are fructose-rich soluble and highly fermentable fibers with simple structures (long chains, some with branches–like the fructose equivalent of cellulose). Shorter chain fructans are called fructooligosaccharides, whereas longer chain fructans are called inulins.
In a systematic review and meta-analysis of 64 different fiber studies, fructan consumption (along with galacto-oligosaccharide consumption) was associated with significantly greater abundance of the very important probiotics Bifidobacterium and Lactobacillus compared to other fiber types. The microbial effects of inulin have also been studied extensively, with various research showing that inulin increases levels of the beneficial butyrate producing bacteria.
Inulin fiber is one of the most heavily studied functional fibers. They are naturally occurring in a variety of plants including chicory, onions, and Jerusalem artichoke.
Resistant starch
Resistant starch is really starch and doesn’t fit the original technical definition of fiber, which was limited to plant cell wall constituents. Resistant starch is considered to be a fiber because amylase, the enzyme that breaks starch into individual glucose units, doesn’t work on this type of starch. Resistant starch is insoluble yet highly fermentable, and plays a major role in the generation of short-chain fatty acids by gut bacteria. Further, resistant starch is divided into four subgroups: RS1, which is physically inaccessible due to being bound within cell walls; RS2, which is tightly packed ungelatinized granules found in certain raw starchy foods; RS3 (retrograded amylose), which is formed when certain starchy foods are cooked and then cooled down; and RS4, which is formed via a manmade chemical process.
In general, resistant starch is famous for feeding short-chain fatty acid-producing bacteria and enhancing levels of butyric acid. But different forms of resistant starch can have significantly different impacts on the gut microbiome, due fermentation preferences of various bacteria and variations in the speed of fermentation of the resistant starch subclasses.
Sources of resistant starch include grains, legumes, and seeds for RS1; green bananas, green plantains, and raw potato starch for RS2; cooked and cooled potatoes and cooked and cooled rice for RS3; and enzymatically or chemically modified starches sold under various brand names for RS4.
Want to Know ALL the Easy Steps to Nutrivore?
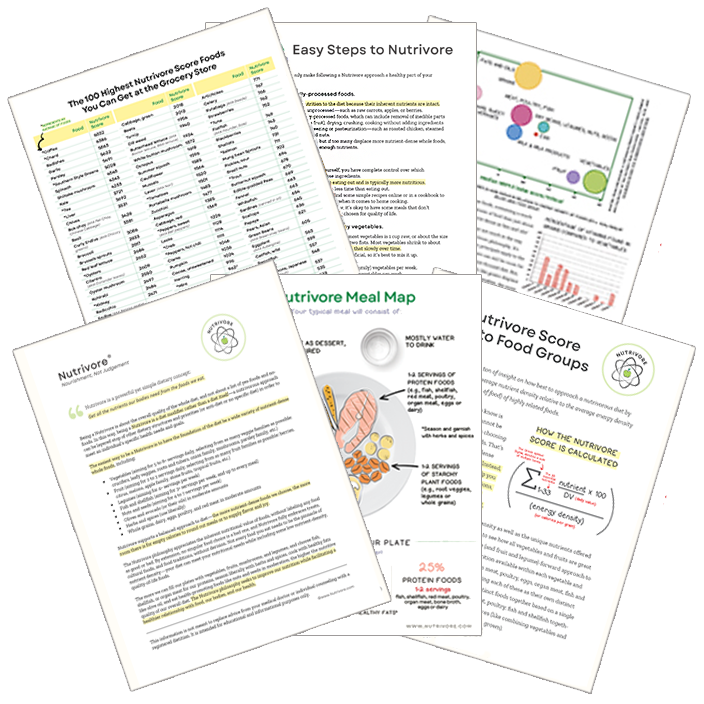
Get it Directly in Your Inbox!
The Nutrivore Newsletter is a weekly email that delivers bite-size fun facts, practical tips, recipes and resources. Sign up now and get 5 free guides directly to your inbox:
- Easy Steps to Nutrivore 4-page guide
- Nutrivore Foundational Foods 6-page guide
- Nutrivore Score Guide to Food Groups 3-page guide
- Nutrivore Meal Map
- Top 100 Nutrivore Score Foods
Benefits of Whole Food Fiber Sources
Even among fibers of the same class, differences in the fine-level structure can impact the interaction with the microbiota. For example, the cellulose in an apple peel is different than the cellulose in cabbage, and this may have a slightly different effect in your digestive tract.
Researchers have pointed to the idea of unique chemical arrangements called “discrete structures” that exist within fiber molecules, and which align with encoded gene clusters in bacterial genomes. These discrete carbohydrate structures (which likely number in the thousands!) provide nutrient niches for bacteria, triggering the expression of bacterial enzymes depending on the “cues” contained within the unique fiber structure. The huge number of potential fiber structures is due to a combination of basic differences in fiber types, variations in genotype and growing environment of the plants, subsets of structures within polymers, linkage types, alterations in fiber taken from different anatomical parts of the plant, carbohydrate chain length, and particle size—among other factors! As a consequence, fibers that vary even in seemingly minor ways might favor fermentation by bacteria down to the strain level, creating highly complex interactions.
For this reason, it is vastly preferable to get your dietary fiber from whole and minimally-processed foods, which naturally contain a wide diversity of fiber types that feed a wide diversity of gut microbes. When a single fiber type is isolated and used as a supplement or food additive, you miss out on the ability to support a diverse community of microbes within your gut.
How Much Fiber Do We Need?
The recommended dietary intake for fiber is 14 grams per 1000 kcal. So, if you eat a 2,000 calorie per day diet, aim for at least 28 grams of fiber. Fiber is one of those “the more, the better” nutrients. For example, a 2015 meta-analysis calculated that, for every 10 grams of daily fiber you consume, risk of all-cause mortality decreased by 10%.
That being said, if you’re looking to up your fiber intake, it’s best to do so gradually! This is because a sudden increase in fiber intake can cause a variety of unpleasant symptoms, such as gas, bloating, constipation and diarrhea—your gut microbiome needs time to adjust to your dietary changes. It’s recommended to step up your fiber intake weekly, giving your digestive tract a full week to adjust to each incremental increase, until you hit your target fiber intake levels—the recommended amount to step up each week is 5 grams per day. So, if you’re starting out at 10 grams of fiber daily, go up to 15 grams for all of next week, the week after go up to 20 grams for the whole week, and the week after that, go up to 25 grams for the whole week. And, if a 5-gram incremental increase causes gastrointestinal symptoms, it’s okay to take smaller steps and give more time between each increase. It’s also helpful to make sure your fiber is coming from a variety of sources (not from supplements or fiber-fortified meal replacement products like protein bars) and that you’re incorporating some fermented foods, like sauerkraut, yogurt, kefir and kombucha. When in doubt, consult your doctor.
Nutrient Daily Values
Nutrition requirements and recommended nutrient intake for infants, children, adolescents, adults, mature adults, and pregnant and lactating individuals.
The Top 5 Foods for the Top 5 Nutrient Shortfalls
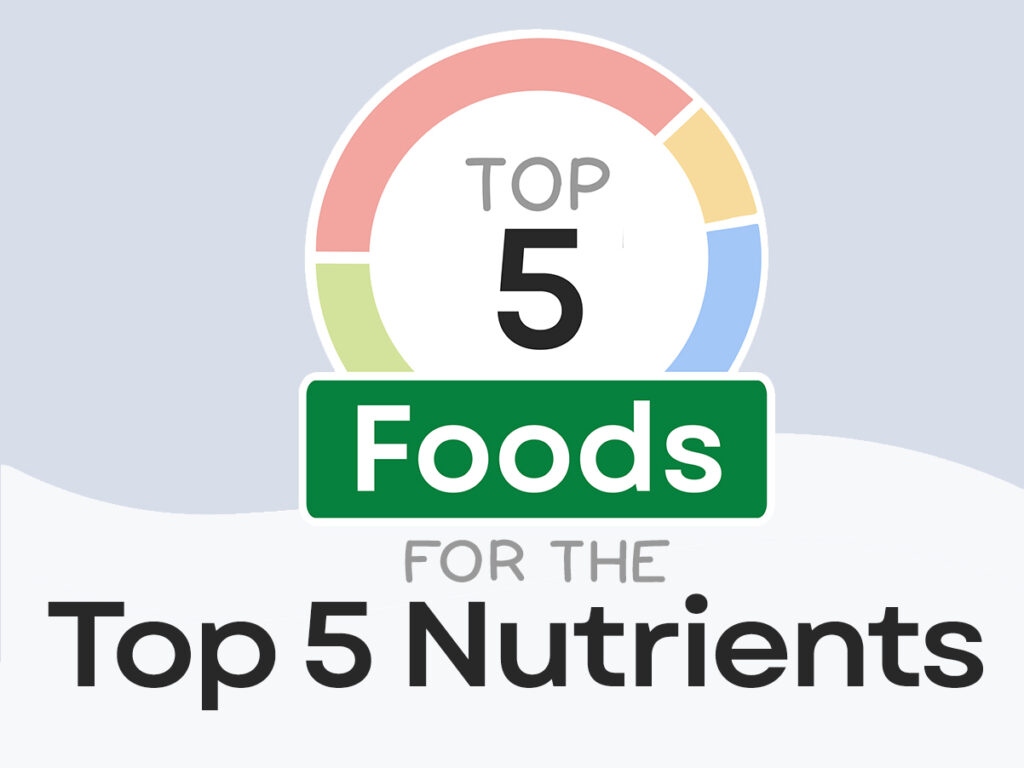
Get it Directly in Your Inbox!
Sign up to get 7 awesome e-mails over the next three weeks to learn about the five most common dietary nutrient shortfalls, how they impact your health, and the top foods to eat (and some yummy recipes to get you started) to up your intake of these incredibly important nutrients!
Good Food Sources of Fiber
The following foods are also excellent or good sources of fiber, containing at least 2.8 grams per serving.
Easily track your servings of Nutrivore Foundational Foods!
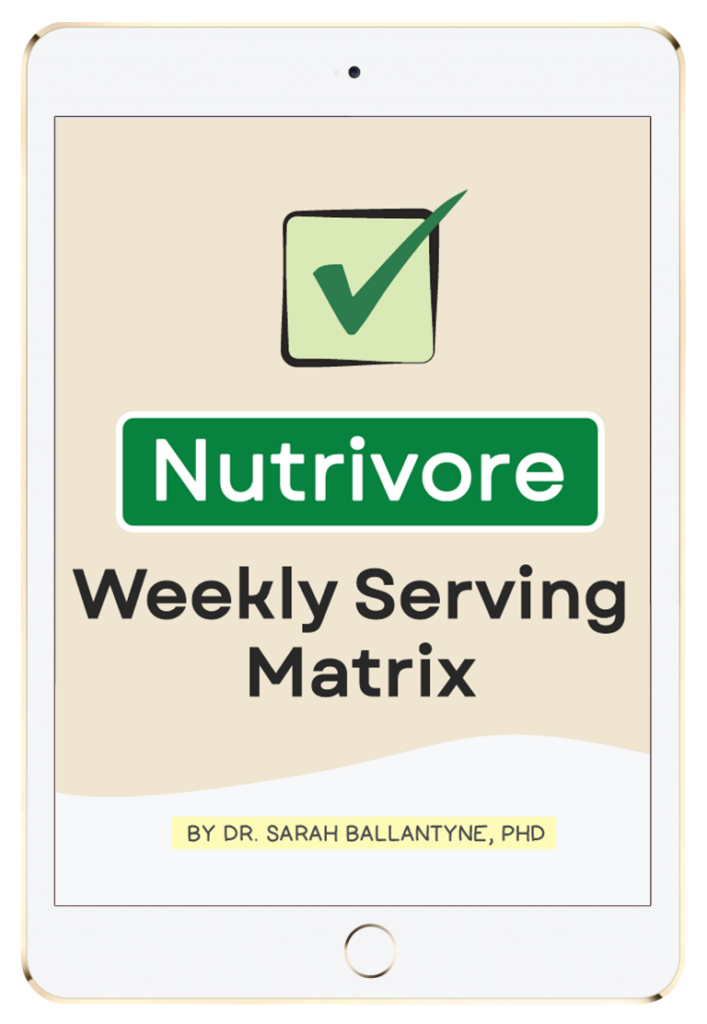
The Nutrivore Weekly Serving Matrix
The Nutrivore Weekly Serving Matrix digital resource is an easy-to-use and flexible weekly checklist designed to help you maximize nutrient-density and meet serving suggestions of Nutrivore foundational foods, all without having to weigh or measure your foods!
Includes a 22-page instructional guide and downloadable interactive guides.
Buy now for instant digital access.
Citations
Expand to see all scientific references for this article.
Bamberger, C., A. Rossmeier, K. Lechner, et al. 2018. “A Walnut-Enriched Diet Affects Gut Microbiome in Healthy Caucasian Subjects: A Randomized, Controlled Trial.” Nutrients. 10(2):244. doi:10.3390/nu10020244.
Banno, T., Y. Komori, S. Suzuki, K. Tanabe, S. Kasaoka, Y. Benno. 2016. “Sweet Potato Improves Defecation and Gut Microbiota of Female University Students.” Nippon Eiyo Shokuryo Gakkaishi. 69(5):229-235. doi:10.4327/jsnfs.69.229.
Benno, Y., K. Endo, H. Miyoshi, T. Okuda, H. Koishi, and T. Mitsuoka. 1989. “Effect of Rice Fiber on Human Fecal Microflora.” Microbiology and Immunology. 33:435-440. doi:10.1111/j.1348-0421.1989.tb01992.x.
Bibas Bonet, M.E., O. Meson, A. de Moreno de LeBlanc. 2010. “Prebiotic effect of yacon (Smallanthus sonchifolius) on intestinal mucosa using a mouse model.” Food and Agricultural Immunology. 21(2):175-189. doi:10.1080/09540100903563589.
Blaiotta, G., B. La Gatta, M. Di Capua, A. Di Luccia, R. Coppola, M. Aponte. 2013. “Effect of chestnut extract and chestnut fiber on viability of potential probiotic Lactobacillus strains under gastrointestinal tract conditions.” Food Microbiology. 36(2):161-9. doi:10.1016/j.fm.2013.05.002.
Blair, B., and M. Lamb. 2017. “Evaluating Concentrations of Pesticides and Heavy Metals in the U.S. Peanut Crop in the Presence of Detection Limits.” Peanut Science. 44. doi:10.3146/PS17-6.1.
Bussolo de Souza, C., G. Roeselers, F. Troost, D. Jonkers, M.E. Koenen, and K. Venema. 2014. “Prebiotic effects of cassava bagasse in TNO’s in vitro model of the colon in lean versus obese microbiota.” Journal of Functional Foods. 11:210-220. doi:10.1016/j.jff.2014.09.019.
Byerley, L.O., D. Samuelson, E. Blanchard 4th, et al. 2017. “Changes in the gut microbial communities following addition of walnuts to the diet.” Journal of Nutritional Biochemistry. 48:94‐102. doi:10.1016/j.jnutbio.2017.07.001.
Caetano, B.F., N.A. de Moura, A.P. Almeida, M.C. Dias, K. Sivieri, and L.F. Barbisan. 2016. “Yacon (Smallanthus sonchifolius) as a Food Supplement: Health-Promoting Benefits of Fructooligosaccharides.” Nutrients. 8(7):436. doi:10.3390/nu8070436.
Campos, D., I. Betalleluz-Pallardel, R. Chirinos A. Aguilar-Galvez, G. Noratto and R. Pedreschi. 2012. “Prebiotic effects of yacon (Smallanthus sonchifoliusPoepp. & Endl), a source of fructooligosaccharides and phenolic compounds with antioxidant activity.” Food Chemistry. 135(3):592-1599.
Carlson, J., T. Gould, and J. Slavin.2106. “In vitro analysis of partially hydrolyzed guar gum fermentation on identified gut microbiota.” Anaerobe. 42:60-66. doi:10.1016/j.anaerobe.2016.08.006.
Carmody, R.N., G.S. Weintraub, and R.W. Wrangham. 2011. “Energetic consequences of thermal and nonthermal food processing.” Proceedings of the National Academy of Sciences. 108(48):19199-19203. doi:10.1073/pnas.1112128108.
Chang, M.C. and W.C. Morris. 1990. “The Effect of Heat Treatments on Dietary Fiber as Assessed by Scanning Electron Microscopy.” Journal of Food Processing and Preservation. 14:335-343. doi:10.1111/j.1745-4549.1990.tb00138.x.
Chutkan R, Fahey G, Wright WL, McRorie J. Viscous versus nonviscous soluble fiber supplements: mechanisms and evidence for fiber-specific health benefits. J Am Acad Nurse Pract. 2012 Aug;24(8):476-87. doi: 10.1111/j.1745-7599.2012.00758.x.
Connolly, M.L., K.M. Tuohy, and J.A. Lovegrove. 2012. “Wholegrain Oat-Based Cereals Have Prebiotic Potential and Low Glycaemic Index.” British Journal of Nutrition. 108(12):2198–2206. doi:10.1017/S0007114512000281.
Davison, K.M. and N.J. Temple. 2018. “Cereal fiber, fruit fiber, and type 2 diabetes: Explaining the paradox.” Journal of Diabetes and its Complications.” 32(2):240-245. doi:10.1016/j.jdiacomp.2017.11.002.
D’hoe, K., L. Conterno, F. Fava, et al. 2018. “Prebiotic Wheat Bran Fractions Induce Specific Microbiota Changes.” Frontiers in Microbiology. 9(31). doi:10.3389/fmicb.2018.00031.
Eslinger, A.J., L.K. Eller, R.A. Reimer. 2014. ”Yellow pea fiber improves glycemia and reduces Clostridium leptum in diet-induced obese rats.” Nutrition Research. 34(8):714-22. doi:10.1016/j.nutres.2014.07.016.
Gamage, H.K.A.H., Tetu, S.G., Chong, R.W.W. et al. 2017. “Cereal products derived from wheat, sorghum, rice and oats alter the infant gut microbiota in vitro.” Scientific Reports. 7(14312). doi:10.1038/s41598-017-14707-z.
Geyer, M., I. Manrique, L. Degen, and C. Beglinger. 2008. “Effect of Yacon (Smallanthus sonchifolius) on Colonic Transit Time in Healthy Volunteers.” Digestion. 78:30-33. doi: 10.1159/000155214.
Govers, M.J., N.J. Gannon, F.R. Dunshea, P.R. Gibson, and J.G. Muir. 1999. “Wheat bran affects the site of fermentation of resistant starch and luminal indexes related to colon cancer risk: a study in pigs.” Gut. 45(6):840‐847. doi:10.1136/gut.45.6.840.
Guan, G., H. Wang, S. Chen, et al. 2016. “Dietary Chitosan Supplementation Increases Microbial Diversity and Attenuates the Severity of Citrobacter rodentiumInfection in Mice.” Mediators of Inflammation. 2016(9236196). doi:10.1155/2016/9236196.
Guevara-Arauza, J.C., J. de Jesús Ornelas-Paz, D.J. Pimentel-González, et al. 2012. “Prebiotic effect of mucilage and pectic-derived oligosaccharides from nopal (Opuntia ficus-indica).” Food Science and Biotechnology. 21:997–1003. doi:10.1007/s10068-012-0130-1.
Gullón, B., P. Gullón, F.K. Tavaria, and R. Yáñez. 2016. “Assessment of the prebiotic effect of quinoa and amaranth in the human intestinal ecosystem.” Food & Function. 7(9):3782-3788. doi:10.1039/C6FO00924G.
Hafidah, A., E. Sulistyaningsih, W. Handayani, and A.A.I. Ratnadewi. 2018. “Prebiotic potential of xylooligosaccharides derived from cassava dregs in Balb/c mice colon.” Pertanika Journal of Tropical Agricultural Science. 41(3):1021-1031.
Hamaker, B.R., and Y.E. Tuncil. 2014. “A Perspective on the Complexity of Dietary Fiber Structures and Their Potential Effect on the Gut Microbiota.” Journal of Molecular Biology. 426(23):3838-3850. doi:10.1016/j.jmb.2014.07.028
Hashemi, Z., J. Fouhse, H.S. Im, C.B. Chan, and B.P. Willing. 2017. “Dietary Pea Fiber Supplementation Improves Glycemia and Induces Changes in the Composition of Gut Microbiota, Serum Short Chain Fatty Acid Profile and Expression of Mucins in Glucose Intolerant Rats.” Nutrients. 9(11):1236. doi:10.3390/nu9111236.
Helou, C., S. Denis, M. Spatz, et al. 2015. “Insights into bread melanoidins: fate in the upper digestive tract and impact on the gut microbiota using in vitro systems.” Food & Function. 6(12):3737-45. doi:10.1039/c5fo00836k.
Hernández-Alonso, P., D. Cañueto, S. Giardina, et al. 2017. “Effect of pistachio consumption on the modulation of urinary gut microbiota-related metabolites in prediabetic subjects.” The Journal of Nutritional Biochemistry. 45:48-53. doi:10.1016/j.jnutbio.2017.04.002.
Hernández-Alonso, P., M. Bulló, and J. Salas-Salvadó. 2016. “Pistachios for Health.” Nutrition Today. 51(3):133-138. doi:10.1097/NT.0000000000000160.
Holscher, H.D. 2017. “Dietary fiber and prebiotics and the gastrointestinal microbiota.” Gut Microbes. 8(2):172‐184. doi:10.1080/19490976.2017.1290756.
Humblot, C., A. Bruneau, M. Sutren, et all. 2005. “Brussels sprouts, inulin and fermented milk alter the faecal microbiota of human microbiota-associated rats as shown by PCR-temporal temperature gradient gel electrophoresis using universal, Lactobacillus and Bifidobacterium 16S rRNA gene primers.” British Journal of Nutrition. 93(5):677-684. doi:10.1079/BJN20051372.
Jenkins, D.J., C.W. Kendall, V. Vuksan, et al. 1999. “The effect of wheat bran particle size on laxation and colonic fermentation.” Journal of the American College of Nutrition. 18(4):339-45. doi:10.1080/07315724.1999.10718873.
Kaczmarek, J.L., X. Liu, and C.S. Charron. 2019. “Broccoli consumption affects the human gastrointestinal microbiota.” Journal of Nutritional Biochemistry. 63:27-34. doi: 10.1016/j.jnutbio.2018.09.015.
Kashyap, P.C., A. Marcobal, L.K. Ursell, et al. 2013. “Complex interactions among diet, gastrointestinal transit, and gut microbiota in humanized mice.” Gastroenterology. 144(5):967‐977. doi:10.1053/j.gastro.2013.01.047.
Kellingray, L., H.S. Tapp, S. Saha, J.F. Doleman, A. Narbad, and R.F. Mithen. 2017. “Consumption of a diet rich in Brassica vegetables is associated with a reduced abundance of sulphate-reducing bacteria: A randomised crossover study.” Molecular Nutrition & Food Research. 61(9):1600992. doi:10.1002/mnfr.201600992.
Kim, J.Y., Y.M. Kwon, I.S. Kim, et al. 2018. “Effects of the Brown Seaweed Laminaria japonica Supplementation on Serum Concentrations of IgG, Triglycerides, and Cholesterol, and Intestinal Microbiota Composition in Rats.” Frontiers in Nutrition. 5(23). doi:10.3389/fnut.2018.00023.
Kopf, J.C., M.J. Suhr, J. Clarke, et al. 2018. “Role of whole grains versus fruits and vegetables in reducing subclinical inflammation and promoting gastrointestinal health in individuals affected by overweight and obesity: a randomized controlled trial.” Nutrition Journal. 17(1):72. doi:10.1186/s12937-018-0381-7.
Kurdi, P., and C. Hansawasd. “Assessment of the prebiotic potential of oligosaccharide mixtures from rice bran and cassava pulp.” LWT – Food Science and Technology. 63(2):1288-1293. doi.org/10.1016/j.lwt.2015.04.031.
Lamuel-Raventos, R.M., and M.S. Onge. 2017. “Prebiotic nut compounds and human microbiota.” Critical Reviews in Food Science and Nutrition. 57(14):3154‐3163. doi:10.1080/10408398.2015.1096763.
Lappi, J., J. Salojärvi, M. Kolehmainen, et al. 2013. “Intake of Whole-Grain and Fiber-Rich Rye Bread Versus Refined Wheat Bread Does Not Differentiate Intestinal Microbiota Composition in Finnish Adults with Metabolic Syndrome.” The Journal of Nutrition. 143(5):648–655. doi:10.3945/jn.112.172668.
Lestari, Y., I. Kusharto, C.M. Rimbawan. 2013. “The Effect of Feeding Diet Containing Functional Biscuit Enriched with Catfish (Clarias gariepinus), Sweet Potatoes (Ipomoea sp.) Flours and Probiotic Enterococcus faecium IS-27526 Cream on the Profile of Fecal Microbiota of Female Aged Sprague Dawley Rats.” Scientific Repository.
Lin, F., M. Hasegawa and O. Kodama. 2003. “Purification and Identification of Antimicrobial Sesquiterpene Lactones from Yacon (Smallanthus sonchifolius) Leaves.” Bioscience, Biotechnology, and Biochemistry. 67(10):2154-2159. doi:10.1271/bbb.67.2154.
Liu, G., L. Xiao, T. Fang, et al. 2014. “Pea fiber and wheat bran fiber show distinct metabolic profiles in rats as investigated by a 1H NMR-based metabolomic approach.” 10(3):e0119117. PLoS One. 9(12):e115561. doi:10.1371/journal.pone.0115561.
Liu, W., Y. Zhang, B. Qiu, et al. 2018. “Quinoa whole grain diet compromises the changes of gut microbiota and colonic colitis induced by dextran Sulfate sodium in C57BL/6 mice.” Scientific Reports. 8(14916). doi:10.1038/s41598-018-33092-9.
Liu, X., Y. Wang, J.L. Hoeflinger, B.P. Neme, E.H. Jeffery, M.J. Miller. 2017. “Dietary Broccoli Alters Rat Cecal Microbiota to Improve Glucoraphanin Hydrolysis to Bioactive Isothiocyanates.” Nutrients. 9(3):262. doi:10.3390/nu9030262.
Liu, Z., W. Wang, G. Huang, W. Zhang, and L. Ni. 2016. “In vitro and in vivo evaluation of the prebiotic effect of raw and roasted almonds (Prunus amygdalus).” Journal of the Science of Food and Agriculture. 96(5):1836‐1843. doi:10.1002/jsfa.7604.
Liu, Z., W. Wang, G. Huang, W. Zhang, and L. Ni. 2016. “In vitro and in vivo evaluation of the prebiotic effect of raw and roasted almonds (Prunus amygdalus).” Journal of the Science of Food and Agriculture.96(5):1836‐1843. doi:10.1002/jsfa.7604.
Liu, Z., X. Lin, G. Huang, W. Zhang, P. Rao, L. Ni. 2014. “Prebiotic effects of almonds and almond skins on intestinal microbiota in healthy adult humans.” Anaerobe. 26:1-6. doi:10.1016/j.anaerobe.2013.11.007.
Maccaferri, S., A. Klinder, S. Cacciatore, et al. 2012. “In vitro fermentation of potential prebiotic flours from natural sources: Impact on the human colonic microbiota and metabolome.” Molecular Nutrition & Food Research. 56:1342-1352. doi:10.1002/mnfr.201200046.
Maier, T.V., M. Lucio, L.H. Lee. 2017. “Impact of Dietary Resistant Starch on the Human Gut Microbiome, Metaproteome, and Metabolome.” mBio. 8(5)e01343-17. doi:10.1128/mBio.01343-17.
Mandalar,i G., C. Nueno-Palop, G. Bisignano, M.S. Wickham, and A. Narbad. 2008. “Potential prebiotic properties of almond (Amygdalus communis L.) seeds.” Applied Environmental Microbiology. 74(14):4264‐4270. doi:10.1128/AEM.00739-08.
Mandalari, G., R.M. Faulks, C. Bisignano, K.W. Waldron, A. Narbad, and M.S. Wickham. 2010. “In vitro evaluation of the prebiotic properties of almond skins (Amygdalus communis L.).” FEMS Microbiology Letters, 304:116-122. doi:10.1111/j.1574-6968.2010.01898.x.
Martínez, I., J. Kim, P.R. Duffy, V.L. Schlegel, J. Walter. 2010. “Resistant starches types 2 and 4 have differential effects on the composition of the fecal microbiota in human subjects.” PLoS One. 5(11):e15046. doi:10.1371/journal.pone.0015046.
Martínez, I., J.M. Lattimer, K.L. Hubach, et al. 2013. “Gut microbiome composition is linked to whole grain-induced immunological improvements.” The ISME Journal. 7(2):269‐280. doi:10.1038/ismej.2012.104.
Martins, J.F.L., V.C. Rafael, E.A. Filomena, F.C. Rodrigues, S.S.R. Sartori, and C.L.L.F. Ferreira. 2016. “Product based yacon and modulation of microbiota, fatty acid profile and lipid in ovariectomized female rats.” Brazilian Journal of Nutrition. 29(5). doi.org/10.1590/1678-98652016000500009.
Muchiri, M., and A.L. McCartney. 2017. “In vitro investigation of orange fleshed sweet potato prebiotic potential and its implication on human gut health.” Functional Food in Health & Disease. 710) doi:10.31989/ffhd.v7i10.361.
Narbad, A., and J.T. Rossiter. 2018. “Gut Glucosinolate Metabolism and Isothiocyanate Production.” Molecular Nutrition & Food Research. 62(18). doi:10.1002/mnfr.201700991.
Ohashi, Y., K, Sumitani, M. Tokunaga, N. Ishihara, T. Okubo, and T. Fujisawa. 2015. “Consumption of partially hydrolysed guar gum stimulates Bifidobacteria and butyrate-producing bacteria in the human large intestine.” Beneficial Microbes. 6(4):451-455. doi:10.3920/BM2014.0118.
Osundahunsi, O., A. Williams and I. Oluwalana. 2011. “Prebiotic effects of cassava fibre as an ingredient in cracker-like products.” Food & Function. 3:159-63. doi:10.1039/c1fo10183h.
Parussolo, G., R.T. Busatto, J. Schmitt, R. Pauletto, P.F. Schons, and E.F. Ries. “Synbiotic ice cream containing yacon flour and Lactobacillus acidophylus NCFM.” LWT – Food Science and Technology. 82:192-198. doi:10.1016/j.lwt.2017.04.049.
Pérez-Burillo, S., S. Pastoriza, N. Jimenez-Hernandez, G. D’Auria, M.P. Francino, and J.A. Rufian-Henares. 2018. “Effect of Food Thermal Processing on the Composition of the Gut Microbiota.” Journal of Agricultural and Food Chemistry. 66(43)11500-11509. doi.org/10.1021/acs.jafc.8b04077.
Queiroz-Monici, K.S., G.E.A. Costa, N. Silva, S.M.P.M. Reis, and A.C. de Oliveira. 2005. “Bifidogenic effect of dietary fiber and resistant starch from leguminous on the intestinal microbiota of rats.” Nutrition. 21(5):602-608. doi:10.1016/j.nut.2004.09.019.
Roager, H.M., J.K. Vogt, M. Kristensen, et al. 2019. “Whole grain-rich diet reduces body weight and systemic low-grade inflammation without inducing major changes of the gut microbiome: a randomised cross-over trial.” Gut. 68:83-93. doi:10.1136/gutjnl-2017-314786.
Rose, D.J. 2014. “Impact of Whole Grains on the Gut Microbiota: the next Frontier for Oats?” British Journal of Nutrition. 112(S2):S44–S49. doi:10.1017/S0007114514002244.
Sargautiene, V., Z. Zariņš, and R. Ligere. 2015. “Effects of the Modulation Gut Microbiota by Oat Beta Glucan on Type 2 Diabetes Mellitus.” International Journal of Research Studies in Biosciences. 3(5):201-208. Doi:
Schlörmann, W., and M. Glei. 2017. “Potential health benefits of β-glucan from barley and oat.” Science & Research. 64(10):145–149. doi:10.4455/eu.2017.039.
Simpson, H.L. and B.J. Campbell. 2015. “Review article: dietary fibre–microbiota interactions.” Alimentary Pharmacology and Therapeutics. 42:158-179. doi:10.1111/apt.13248.
So, D., K. Whelan, M. Rossi. 2018. “Dietary fiber intervention on gut microbiota composition in healthy adults: a systematic review and meta-analysis.” The American Journal of Clinical Nutrition. 1076):965–983. doi:10.1093/ajcn/nqy041.
Sonia, S., F. Witjaksono, and R. Ridwan. 2015. “Effect of cooling of cooked white rice on resistant starch content and glycemic response.” Asia Pacific Journal of Clinical Nutrition. 24(4):620-5. doi:10.6133/apjcn.2015.24.4.13.
Tang, C., J. Sun, B. Zhou, et al. 2018. “Effects of polysaccharides from purple sweet potatoes on immune response and gut microbiota composition in normal and cyclophosphamide treated mice.” Food & Function. 9(2):937-950. doi:10.1039/c7fo01302g.
Tian, S., X. Liu, P. Lei, X. Zhang, and Y. Shan. 2018. “Microbiota: a mediator to transform glucosinolate precursors in cruciferous vegetables to the active isothiocyanates”. Journal of the Science of Food and Agriculture. 98:1255-1260. doi:10.1002/jsfa.8654.
Ukhanova, M., X. Wang, D.J. Baer, J.A. Novotny, M. Fredborg, and V. Mai. 2014. “Effects of Almond and Pistachio Consumption on Gut Microbiota Composition in a Randomised Cross-over Human Feeding Study.” British Journal of Nutrition. 111(12):2146–2152. doi:10.1017/S0007114514000385.
Uyeno, Y., S. Katayama, and S. Nakamura. 2014. “Changes in mouse gastrointestinal microbial ecology with ingestion of kale.” Beneficial Microbes. 5(3):345-349. doi:10.3920/BM2013.0073.
van den Brandt, P.A., and L. J. Schouten, 2015. “Relationship of tree nut, peanut and peanut butter intake with total and cause-specific mortality: a cohort study and meta-analysis.” International Journal of Epidemiology. 44(3):1038–1049. doi:10.1093/ije/dyv039.
Vandeputte, D., G. Falony, S. Vieira-Silva, et al. 2017. “Prebiotic inulin-type fructans induce specific changes in the human gut microbiota.” Gut. 66(11):1968-1974. doi:10.1136/gutjnl-2016-313271.
Vanegas, S.M., M.Meydani, J.B. Barnett, et al. 2017. “Substituting whole grains for refined grains in a 6-wk randomized trial has a modest effect on gut microbiota and immune and inflammatory markers of healthy adults.” The American Journal of Clinical Nutrition. 105(3):635–650. doi:10.3945/ajcn.116.146928.
Varo, P., R. Laine, and P. Koivistoinen. 1983. “Effect of heat treatment of dietary fiber: interlaboratory study.” Journal Association of Official Analytical Chemists. 66(4):933-8. doi:10.1093/jaoac/66.4.933.
Velez, E., N. Castillo, O. Mesón, A. Grau, M.E. Bibas Bonet, and G. Perdigón. 2013. “Study of the Effect Exerted by Fructo-Oligosaccharides from Yacon (Smallanthus Sonchifolius) Root Flour in an Intestinal Infection Model with Salmonella Typhimurium.” British Journal of Nutrition. 109(11):1971–79. doi:10.1017/S0007114512004230.
Venkataraman, A., J.R. Sieber, A.W. Schmidt, C. Waldron, K.R. Theis, T.M. Schmidt. 2016. “Variable responses of human microbiomes to dietary supplementation with resistant starch.” Microbiome. 4(1):33. doi:10.1186/s40168-016-0178-x.
Wang, L., L. Hu, S. Yan, et al. 2017. “Effects of different oligosaccharides at various dosages on the composition of gut microbiota and short-chain fatty acids in mice with constipation.” Food & Function. 8(5):1966-1978. doi: 10.1039/c7fo00031f.
Yang Y, Zhao LG, Wu QJ, Ma X, Xiang YB. Association between dietary fiber and lower risk of all-cause mortality: a meta-analysis of cohort studies. Am J Epidemiol. 2015 Jan 15;181(2):83-91. doi: 10.1093/aje/kwu257.
Zhang, C., S. Jiao, Z.A. Wang, and Y. Du. 2018. “Exploring Effects of Chitosan Oligosaccharides on Mice Gut Microbiota in in vitro Fermentation and Animal Model.” Frontiers in Microbiology. 9:2388. doi:10.3389/fmicb.2018.02388.
Zhang, L., C. Mu, X. He. 2016. “Effects of dietary fibre source on microbiota composition in the large intestine of suckling piglets.” FEMS Microbiology Letters. 363(14). doi:10.1093/femsle/fnw138.
Zheng, J., G. Cheng, Q. Li, et al. 2018. “Chitin Oligosaccharide Modulates Gut Microbiota and Attenuates High-Fat-Diet-Induced Metabolic Syndrome in Mice.” Marine Drugs. 16(2):66. doi:10.3390/md16020066.