Key Takeaways (expand)
- Choline is an essential, water-soluble, vitamin-like compound that’s similar to B vitamins.
- Some choline can be produced by our bodies, but we can’t synthesize enough to meet all of our metabolic needs, making it necessary to consume it from foods as well!
- Choline is a key component of the phospholipids phosphatidylcholine and sphingomyelin, both of which are essential structural components of the cell membrane.
- Along with their structural roles, choline-derived phospholipids contribute to both the growth and programmed death of cells, and can be broken down to produce additional metabolites with cell-signaling properties.
- Phosphatidylcholine is needed for VLDL production, making choline a major player in lipid transport and metabolism (especially in the liver).
- Choline is needed to produce platelet-activating factor—a powerful mediator of inflammation, shock, and allergic responses.
- Choline is also needed for the production of acetylcholine—a neurotransmitter involved in memory, learning, mood, muscle control, attention, and arousal.
- Choline serves as a precursor to betaine, a molecule that’s needed for the conversion of homocysteine into methionine.
- Choline and betaine are also both methyl donors, giving them roles in methylation reactions.
- Choline can sometimes elevate levels of a potentially harmful compound called trimethylamine-n-oxide (TMAO), which has been associated with higher risk of cardiovascular disease and some other chronic diseases.
- Whether choline increases TMAO levels depends on the composition of the gut microbiota, the source and form of the choline, and potentially other genetic and lifestyle variables.
- Choline has a significant impact on liver health, and may help protect against non-alcoholic fatty liver disease (NAFLD).
- Choline has a complex relationship with cardiovascular health, with studies inconsistently showing positive, neutral, or inverse associations between higher intakes and cardiovascular risk.
- Choline appears to be protective against certain cancers, including breast, colorectal, and liver cancer.
- Choline intake during pregnancy appears to influence the risk of neural tube defects and some aspects of cognition in early childhood.
- Choline may have protective effects for neurological health, including cognitive performance, and risk of dementia or Alzheimer’s disease.
- Some evidence suggests choline may play a role in autism.
- Choline deficiency can lead to excess fat deposition in the liver, muscle damage, fatigue, organ dysfunction, kidney necrosis, increased DNA damage, elevated homocysteine levels, and neurological issues.
- Good sources of choline include egg yolks, poultry, fish, fish eggs, meat (especially liver), cruciferous veggies (like broccoli and cauliflower), salmon, peanuts, soybeans, and dairy.
Table of Contents[Hide][Show]
Choline is a water-soluble, vitamin-like compound that’s similar to B vitamins. It’s essential (meaning it’s needed to sustain life!), and can be obtained both from our diet and through de novo synthesis within the body; however, our bodies generally can’t synthesize enough choline to meet all of our metabolic needs, making it necessary to consume it from foods as well. It was first isolated in 1862, when the German chemist Adolph Strecker isolated it from ox and pig bile. In fact, the word “choline” comes from the greek kholé, meaning “bile”! It wasn’t until 1998 that this amazing nutrient became recognized as essential.
Choline plays important structural roles in cell membranes, and is also involved in cell signaling (through its incorporation into phospholipids and acetylcholine), nerve impulse transmission, methylation reactions, lipid transport and metabolism, and liver and kidney health. As a result, it has wide-ranging impacts on cardiovascular risk, neurological health, and more!
Good food sources of choline include egg yolks, poultry, fish, fish eggs, meat (especially liver), cruciferous veggies (like broccoli and cauliflower), salmon, peanuts, soybeans, and dairy.
Want to know the top 25 foods for this awesome nutrient?
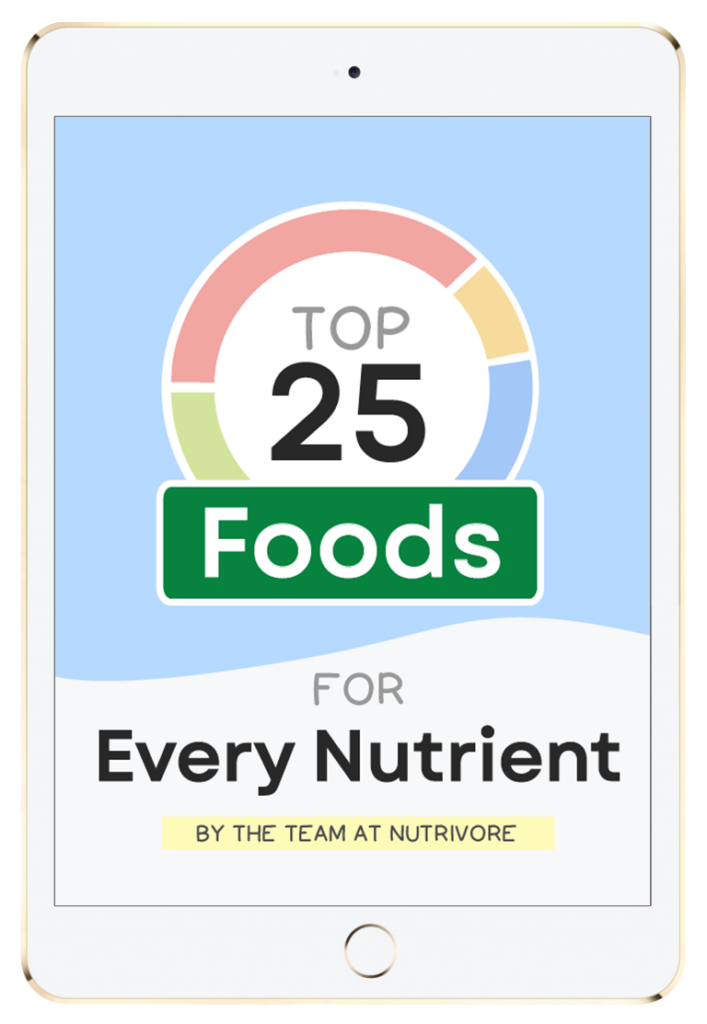
The Top 25 Foods for Every Nutrient
The Top 25 Foods for Every Nutrient e-book is a well-organized, easy-to-use guide to help you choose foods that fit your needs of 42 important nutrients while creating a balanced nutrient-dense diet.
Get two “Top 25” food lists for each nutrient, plus you’ll find RDA charts for everyone, informative visuals, fun facts, serving sizes and the 43 foods that are Nutrient Super Stars!
Buy now for instant digital access.
The Biological Roles of Choline
Many of choline’s roles in the body stem from its presence in cell membranes—AKA the lipid bilayer that protects the inside of the cell from the outside environment. Specifically, choline a key component of the phospholipids phosphatidylcholine and sphingomyelin, both of which are essential structural components of the cell membrane. Sphingomyelin is particularly abundant in the membranes of neurons, though the vast majority (95%!) of choline in the body is in the form of phosphatidylcholine!
These choline-derived phospholipids are incredibly important for cellular function. Along with their structural roles, they contribute to both the growth and programmed death (apoptosis) of cells, and can be broken down to produce additional metabolites with cell-signaling properties (such as ceramide and diacylglycerol).
Through its presence in phosphatidylcholine, choline is also a major player in lipid transport and metabolism. After dietary lipids (fat and cholesterol) reach the liver, they’re packaged into very-low-density lipoproteins (VLDL) in order to be transported through the bloodstream into various tissues throughout the body. One of the compounds needed for VLDL assembly and secretion is phosphatidylcholine. As a result, adequate choline is needed to successfully move lipids out of the liver and prevent them from accumulating there!
Choline also produces other phospholipids with cell-signaling activity, including platelet-activating factor. Platelet-activating factor is a powerful mediator of inflammation, shock, and allergic responses, with an ability to induce platelet aggregation and blood vessel dilation. It also helps regulate respiratory function, and may even influence some components of neurological health, including neurotransmission and synaptic plasticity (the ability of neurons to alter the strength of their connections).
Beyond phospholipids, choline is needed for the production of acetylcholine—a neurotransmitter involved in memory, learning, mood, muscle control, attention, and arousal. Acetylcholine is the primary neurotransmitter for the parasympathetic nervous system (which governs the body’s “rest and digest” processes), where it helps slow heart rate, stimulate digestive processes, and promote relaxation. It was also the very first neurotransmitter shown to regulate circadian rhythms (the body’s sleep-wake cycle), and more recently has been shown to influence the activity of immune cells—giving it a role in immunity!
Choline also serves as a precursor to another notable compound, betaine. Betaine forms from the oxidation of choline within the liver and kidneys, and is needed for the conversion of homocysteine into methionine—in turn helping regulate the homeostasis of these two amino acids. In particular, betaine can help bring down elevated levels of homocysteine, which has been implicated in both cardiovascular and neurological health! It also influences levels of S-adenosylmethionine (SAMe)—a compound made from methionine that’s involved in liver function, the immune system, neurotransmitter synthesis and breakdown, and joint health. Betaine also serves roles in osmoregulation (modulating cell volume), cellular reproduction, liver function, carnitine production, and methylation reactions (due to serving as an important methyl donor).
In some circumstances, choline can also increase levels of a potentially harmful compound called trimethylamine-n-oxide (TMAO). Specifically, certain gut microbes (particularly members of Clostridium, but also members of the bacterial families Deferribacteraceae, Anaeroplasmataceae, Prevotellaceae and Enterobacteriaceae) metabolize choline into trimethylamine (TMA), which enzymes in the liver can further metabolize into TMAO. TMAO, in turn, has been associated with higher risk of some chronic diseases—particularly cardiovascular disease, but also type 2 diabetes, liver disease, and chronic kidney disease. Although these links aren’t fully consistent or conclusive, mechanistic studies suggest that TMAO could alter cholesterol and bile acid metabolism, promote foam cell formation (a component of arterial plaque), and activate inflammatory pathways.
However, whether choline increases TMAO levels depends on a number of individual factors! Most notably, the composition of the gut microbiota (e.g., high or low in TMA-producing bacteria) and the source and form of the choline (e.g., as “free” choline or bound in an ester such as phosphatidylcholine) can significantly influence whether choline ingestion raises TMAO levels in the body. Levels of other nutrients can impact this relationship, too: studies suggest that vitamin D, for example, can inhibit the production of TMA and TMAO from choline by modulating the gut microbiota, even in the presence of high choline intakes.
So, while the choline we consume has the potential to raise our TMAO levels, the link is highly nuanced and variable—and it’s uncertain whether elevated TMAO is causative of disease, or reflective of other processes involved in disease etiology. More research is needed to shine light on this topic!
Lastly, the body can produce a small amount of choline, via the liver phosphatidylethanolamine N-methyltransferase pathway. However, we can’t synthesize enough of it to meet all of our metabolic demands, making dietary choline extremely important for supporting our health!
Interactions with Other Nutrients
Choline metabolism is interconnected with other nutrients—particularly folate, vitamin B12, and methionine. These nutrients are involved in the one-carbon metabolism pathway, where methyl groups are transferred and used for various cellular processes. Adequate levels of folate, vitamin B12, and methionine are crucial for optimal choline metabolism! In addition, folate demand increases when dietary choline supply is low.
Want to Know ALL the Easy Steps to Nutrivore?
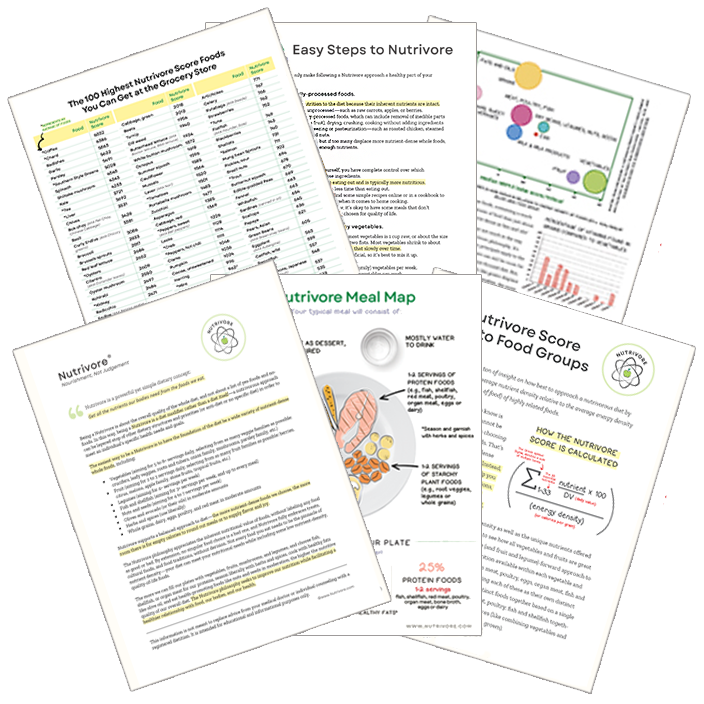
Get it Directly in Your Inbox!
The Nutrivore Newsletter is a weekly email that delivers bite-size fun facts, practical tips, recipes and resources. Sign up now and get 5 free guides directly to your inbox:
- Easy Steps to Nutrivore 4-page guide
- Nutrivore Foundational Foods 6-page guide
- Nutrivore Score Guide to Food Groups 3-page guide
- Nutrivore Meal Map
- Top 100 Nutrivore Score Foods
Choline in Health and Disease
Because it plays so many important roles in human metabolism (particularly cell structure and neurotransmitter synthesis), choline impacts a number of areas of human health—including liver health, cardiovascular health, cancer risk, reproductive health, neurological health, cognition, and more!
Choline and Liver Health
Due to its involvement in the transport and metabolism of fats in the liver, choline has a significant impact on liver health—particularly the prevention of non-alcoholic fatty liver disease (NAFLD). Some research suggests the relationship may be modified by additional factors like gender and BMI.
A 2023 analysis of the National Health and Nutrition Examination Survey (NHANES) data found that optimal choline intake was associated with a 30% lower risk of NAFLD. When the results were divided by gender, the highest versus lowest choline intakes were more strongly protective for women—a 24% lower risk, compared to only 4.5% lower for men.
Likewise, a 2014 study of over 56,000 normal-weight Chinese adults found women in the highest versus lowest quintile of choline intake (412 mg vs. 179 mg daily) had a 32% lower risk of NAFLD. However, after adjusting for other variables, no significant protective effect showed up for overweight women, obese women, or men.
Additionally, a randomized controlled trial from 2012 found that among postmenopausal women with existing NAFLD, inadequate choline intake was associated with increased liver fibrosis (excessive buildup of scar tissue). Interestingly, choline didn’t appear to contribute to disease severity among men, children, or premenopausal women.
And, a 2019 analysis of NHANES data found that BMI strongly modified the association between choline and NAFLD risk, as well as by menopausal status in women. When comparing changes in Fatty Liver Index scores (a surrogate marker for fatty liver disease) for the highest versus lowest quartiles of choline intake, participants with higher BMIs had a score decrease of 11.5, while participants with lower BMIs had a score decrease of only 5.2. Likewise, women over the age of 50 saw a greater risk reduction with higher choline intake compared to other participants: 26% lower versus 14% lower, respectively.
While the effects of chronic choline deficiency on liver health are clear, more research is needed on the effects of suboptimal intakes, especially among different populations!
Choline and Cardiovascular Disease
Choline has a complex relationship with cardiovascular health. On one hand, choline and its metabolites (particularly betaine) are critical for regulating homocysteine levels, with elevated homocysteine being a risk factor for cardiovascular disease. This would suggest a cardio-protective role of choline through the suppression of elevated homocysteine. But, due to its potential to raise TMAO levels, choline has sometimes been associated with increased cardiovascular risk. In general, the research here is inconsistent!
For example, some large, well-designed studies detected no significant relationship between choline and cardiovascular disease incidence and/or mortality. A 2017 meta-analysis of prospective studies, encompassing six studies and over 184,000 participants, found no association between choline or its metabolite, betaine, and cardiovascular disease incidence. A 2022 prospective cohort study likewise found that after adjusting for total energy intake, there was no association between choline or betaine intakes and cardiovascular disease risk over the course of a 10.6-year follow-up. And, a 2015 study of nearly 30,000 Japanese adults found no association between choline intake and death from cardiovascular disease.
In other studies, choline has appeared protective against cardiovascular diseases. For example, a 2023 analysis of NHANES data, including over 14,000 participants, found that people in the highest versus lowest quartile of dietary choline intake had a 31% lower risk of cardiovascular disease—and a 35% lower risk of stroke, in particular! The protective association of choline was strongest for participants who were at least 60 years old, and those with a BMI greater than 30. Additionally, a 2018 analysis of the Jackson Heart Study, following African-American participants for an average of nine years, found a significant 77% reduction in stroke risk with higher dietary choline intake.
However, a 2023 meta-analysis, this one encompassing 17 studies and 33,000 participants, found that people with the highest versus lowest circulating choline concentrations had a 29% higher risk of cardiovascular mortality and a 62% higher risk of all-cause mortality. A 2016 analysis of over 120,000 participants from the Nurses’ Health Study and Health Professionals Follow-up Study found that higher choline consumption (in the form of phosphatidylcholine) was associated with increased cardiovascular mortality, especially among diabetics; for every 100 mg daily increase in phosphatidylcholine intake, the risk rose by 21%.
A 2020 analysis of three prospective cohort studies also found a positive association between choline intake and cardiovascular death, but ethnic background, preexisting health conditions, and some lifestyle variables all seemed to influence the risk. In this study, higher choline intake was associated with increased cardiovascular death among black and Chinese participants, with a weaker link seen among white participants. The risk increase was strongest among people with a history of cardiovascular disease or diabetes. What’s more, alcohol intake seemed to modify the choline-cardiovascular disease relationship, with higher choline intake showing a protective effect against cardiovascular and diabetes-related death among heavy drinkers!
Although the reasons for these contradictory findings aren’t fully known, it’s speculated that TMAO production from choline mediates the choline-cardiovascular disease relationship. Experimental studies have identified possible mechanisms for TMAO in atherosclerosis development and progression, such as increasing oxidative stress, contributing to foam cell formation, increasing platelet aggregation, inducing the release of inflammatory cytokines, and promoting leukocyte adhesion.
Indeed, when both choline and TMAO are assessed in studies, TMAO often appears to be the stronger driver of risk. As one example, a 2014 study of over 3900 stable cardiac patients found that higher plasma choline levels were associated with a 190% greater risk of adverse cardiac events, but only when TMAO levels were also elevated. When TMAO was adjusted for in statistical models, the predictive value of choline for cardiac events disappeared!
Inconsistencies among studies could therefore reflect individual differences in TMAO production from choline. Along with the gut microbiota composition driving these differences, the source and form of the choline itself may also play a role in whether TMAO levels rise in response to higher intake!
For example, a 2020 randomized controlled trial of healthy adult men found that 600 mg of “free” choline (in the form of choline bitartrate) increased blood levels of TMAO three-fold, and urinary TMAO levels 2.5-fold, compared to participants’ baseline levels. However, 600 mg of choline in the form of phosphatidylcholine (the form most commonly found in food) had no effect on TMAO levels. This suggests an important difference in the effects of free choline and lipid-soluble choline on TMAO levels.
A randomized controlled trial from 2021, enrolling healthy adults with normal kidney function, tested the effects of dietary choline from eggs (four daily), phosphatidylcholine supplements, and choline bitartrate supplements. After four weeks, only the choline bitartrate significantly increased participants’ TMAO levels, while the eggs and phosphatidylcholine did not. Likewise, a 2017 crossover trial found no change in TMAO levels from eating three eggs per day for four weeks.
It’s also worth noting that while TMAO has been linked with increased cardiovascular risk in observational studies and has some plausible mechanisms in experiments, whether the relationship is causal in humans is still inconclusive. It’s possible that TMAO may be a marker of cardiovascular risk factors and disrupted metabolic processes, rather than a direct driver of disease.
For example, there’s some evidence that TMAO rises in response to other processes that increase cardiovascular risk, possibly as a way to help the body restore homeostasis. A 2019 paper hypothesized that elevations in TMAO is a beneficial compensatory mechanism to help protect cells from hydrostatic and osmotic stresses that occur during high blood pressure.
Rodent studies have likewise shown that TMAO supplementation extends the lifespan of animals with heart failure, mimicking the effects of heart failure treatments by removing excess salt and water (in turn reducing pressure on the heart). In hypertensive rats, TMAO has also been shown to reduce diastolic dysfunction and heart fibrosis.
In humans, there’s likewise evidence of a blood-pressure-lowering effect of choline via TMAO—suggesting that along with potential mechanisms to harm cardiovascular health, TMAO possesses some protective mechanisms, too. A 2022 population study found an inverse association between total choline intake and blood pressure, with a subsequent rodent experiment confirming a diuretic and blood-pressure-lowering effect specifically of TMAO.
So, what can we take away from these findings? For one, if a relationship does exist between choline intake and adverse cardiovascular outcomes or death, it’s likely mediated by the composition of the gut microbiota, as well as the source and the form of ingested choline (e.g., food or dietary supplements). Likewise, existing cardiometabolic diseases (such as heart disease or diabetes), ethnic background, and other variables may play a role. Even more broadly, it’s worth emphasizing that even when a positive association shows up in studies, it’s unclear whether that association is actually causal.
In general, much more research is needed to fully understand the relationship between choline and cardiovascular health!
Choline and Cancer
Choline appears to be protective for both overall cancer risk, and for a number of specific cancer types—with the exception of prostate cancer, which has sometimes shown an increased risk.
A 2016 meta-analysis of epidemiological studies, encompassing over 513,000 participants, found that those with the highest versus lowest intake of choline had a 18% lower risk of cancer. Likewise, for every 100 mg per day increase in choline plus betaine intake, cancer incidence dropped by 11%!
When it comes to the relationship between choline (and its metabolites) and breast cancer risk, the evidence is mixed. A 2023 meta-analysis found no effect of choline consumption on breast cancer risk in prospective cohort studies, but did find a 62% risk reduction when comparing highest versus lowest choline intakes in case-control studies. For example, a 2013 case-control study of Chinese women found that those in the highest versus lowest quartile of choline intake had a 60% lower risk of breast cancer, and other choline-related compounds (betaine, choline from glycerophosphocholine, phosphocholine, phosphatidylcholine, and sphingomyelin) were all likewise inversely associated with breast cancer risk. However, this protective association was mostly limited to participants with low folate intake. It’s possible that associations between dietary choline and breast cancer risk may modified by folate intake, explaining some of the inconsistent findings on this topic. More research is needed!
A 2015 case-control study of Chinese adults found that those in the highest versus lowest quartile of choline intake had a 46% lower risk of developing colorectal cancer. Reduced risk was also seen with several choline-containing compounds—particularly phosphatidylcholine, glycerophosphocholine, and sphingomyelin. A 2023 case-control study in Italy similarly found that those in the highest versus lowest tertile of choline intake had a 15% reduction in colorectal cancer risk!
Choline intake has also demonstrated a protective effect against liver cancer. In a 2017 case-control study, patients in the highest versus lowest tertile of choline intake had a whopping 66% lower risk of primary liver cancer. Choline-derived compounds (glycerophosphocholine, phosphocholine, phosphatidylcholine, and sphingomyelin) all likewise had significant protective associations.
On the other hand, some (but not all) studies have found a relationship between higher choline intake and greater risk of prostate cancer. A 2012 prospective analysis of nearly 48,000 men from the Health Professional’s Follow-Up Study found that men in the highest versus lowest quintile of choline intake (509 mg vs. 305 mg daily) had a 70% greater risk of lethal prostate cancer. When only choline intake prior to prostate cancer diagnosis was considered, higher intakes were associated with a 198% greater risk!
Likewise, a smaller 2009 study of Swedish men found that higher blood levels of choline were associated with a 46% higher risk of prostate cancer. On the other hand, a 2019 nested case-control study of 6,528 men found no relationship between choline intake and either total or lethal prostate cancer risk.
Although the reasons for a potential link here are unclear, prostate cancer cells do appear to have altered choline metabolism, with higher concentrations of choline-containing compounds in malignant cells compared to healthy cells. Likewise, prostate cancer cells have a selective and high uptake of circulating choline; in fact, radiolabeled choline is used by doctors to identify prostate cancer for this very reason! Likewise, a 2015 prospective cohort study found that elevated TMAO levels were associated with a 36% higher risk of prostate cancer, suggesting a choline-prostate cancer link could be mediated by this metabolite. Once again, more research is needed!
Choline and Neural Tube Defects
Studies suggest choline intake during pregnancy may have an overall protective effect against neural tube defects (congenital conditions that result from the incomplete development of the neural tube, which forms the spinal cord, brain, and surrounding tissues).
A 2004 case-control study found that women with the highest versus lowest choline intake around the time of conception (over 498.46 mg daily versus 290.41 mg daily or less) had a 51% lower risk of a neural tube defect-affected pregnancy. When choline, betaine, and methionine intake were all analyzed together, those with the greatest intake of all three nutrients had an 83% lower risk! Further supporting these findings, a 2009 perspective study, including over 180,000 pregnant participants, found that the highest decile of choline intake was associated with a 86% lower risk of neural tube defects.
A 2022 systematic review and meta-analysis further established links between maternal choline intake and neural tube defect risk. Looking at 30 publications in total (12 for the meta-analysis), low maternal choline intake and/or low serum choline was associated with a 36% higher risk of neural tube defects.
Choline and Childhood Cognition
When it comes to choline and child cognition, the research isn’t fully consistent, but there’s evidence to suggest that maternal choline intake and/or choline intake in early childhood could have benefits for specific areas of cognition.
For example, a 2022 systematic review and meta-analysis found that higher choline intake during the second half of pregnancy and early postnatal period, along with higher choline intakes among young children, had favorable effects on children’s attention, memory, and visual-spatial learning! A 2013 study likewise found that higher maternal choline intake during pregnancy (particularly the second trimester) was associated with better visual memory in children at seven years of age. However, a 2012 study found no relationship between maternal choline intake and cognition when children were assessed at age three—suggesting the effects of choline during pregnancy might be more pronounced at different ages.
Clinical trials have also demonstrated a relationship between maternal choline intake and childhood cognitive development. A 2018 randomized controlled trial tested the effects of two supplemental choline doses (930 vs 480 mg daily) taken during the third trimester of pregnancy, and found that the higher dose led to significant improvements in infant processing speed. In addition, longer fetal exposure to even the lower supplemental choline dose was associated with cognitive benefits, suggesting that length of exposure, not just dose, matters!
Choline might even help protect against damage induced by fetal alcohol exposure. A 2021 randomized controlled trial found that among infants born to heavy-drinking mothers, choline supplements during pregnancy helped mitigate cognitive and developmental damage—including protecting against loss of brain volume and improving 12-month recognition memory.
Similarly, a randomized placebo-controlled trial from 2019 found that high-dose choline (2 g daily) during pregnancy helped reduce the damage from prenatal alcohol exposure—including improving physical growth, visual recognition memory, and eyeblink conditioning compared to the control group!
Choline and Autism
There’s some evidence linking choline with autism spectrum disorders, although more research is needed to confirm the nature of the relationship.
A 2013 study found that a majority (60 – 93%) of children with autism spectrum disorders had choline intakes below the adequate intake level. Given the role of choline in methylation reactions and acetylcholine synthesis, these low intakes could potentially contribute to metabolic imbalances that exacerbate autism symptoms.
A 2019 randomized controlled trial of 60 autistic children found that nine months of supplementation with choline (350 mg daily) along with donepezil (a medication that inhibits acetylcholine breakdown) caused improvements in receptive language skills—with improvements persisting for six months after the treatment ended.
A 2023 Mendelian randomization study (a research method that uses genetic variations to investigate cause-and-effect relationships between variables, simulating a randomized controlled trial) found that out of 10 intestinal metabolites analyzed, choline was the only one with a causal relationship to autism, with higher levels reducing autism risk by 7.5%.
Choline and Neurological Health
Through its role in acetylcholine production and DNA synthesis, choline may have protective effects for neurological health—including cognitive function! A 2012 cross-sectional study of nearly 2200 elderly adults found that those with high (versus low) plasma choline concentrations had significantly better cognitive performance, as measured through several different cognitive tests. When low choline levels occurred along with low vitamin B12 levels, poor test performance nearly tripled!
A 2011 analysis of the Framingham Offspring Cohort, encompassing almost 1400 dementia-free adults, found that higher choline intake was associated with better cognitive performance—including a 40% improvement in verbal memory and a 34% improvement in spatial memory. And, a 2019 prospective cohort study, following nearly 2500 dementia-free Finnish men, found that those with the highest versus lowest intakes of choline and phosphatidylcholine had better cognitive performance, particularly in tests assessing frontal and temporal lobe functioning.
A 2021 cohort study likewise found that among elderly adults, choline intakes of 187.06 – 399.5 mg per day were associated with a 40% reduced risk of low cognitive function (classified as scoring in the bottom 25% for learning ability, processing speed, working memory, verbal fluency, and sustained attention), compared to participants consuming less choline. Interestingly, choline intakes above 399.5 mg per day were not associated with cognitive performance improvements. It’s possible that other dietary or lifestyle factors accompanying the highest choline intakes in this population may have offset the beneficial effects of choline on cognition. However, more research would be needed to understand this finding, as well as test whether a nonlinear relationship appears in other populations as well.
Choline, Dementia, and Alzheimer’s Disease
Along with benefits for cognitively healthy adults, choline may be protective against neurodegenerative diseases like dementia and Alzheimer’s disease.
A 2023 study of two cohorts—one consisting of patients with mild cognitive impairment, and one consisting of patients with moderate or severe Alzheimer’s disease—found that compared to healthy controls, patients in these cohorts had lower circulating levels of choline. Low choline was also associated with loss of white matter integrity, higher levels of neuroinflammation, and increased Alzheimer’s disease pathology.
A 2019 prospective cohort study, following nearly 2500 dementia-free Finnish men, found that each 50 mg increase in daily choline intake was associated with 4% lower risk of dementia and a 17% lower risk of Alzheimer’s disease. Similarly, each 50 mg increase in phosphatidylcholine intake was associated with 10% reduction in dementia risk. Analyzed as quartiles of intake, men with the highest versus lowest phosphatidylcholine intakes (greater than 222 mg daily, versus under 144 mg daily) had a 28% lower risk of developing dementia.
A 2022 analysis of the Framingham Heart Study Offspring Cohort, following over 3200 participants for an average of 16.1 years, found that low choline intake (215 – 219 mg daily or less) was associated with a significantly higher risk of dementia and Alzheimer’s disease. As with some of the research on cognitive function, the risk reduction was limited to moderate choline intakes, with the highest intakes (above 517 – 553 mg daily) associating with non-significant increased risk of dementia and Alzheimer’s disease! Although the reason for this nonlinear relationship needs further research to understand, it’s possible that TMAO levels could play a role. Specifically, elevated TMAO has been associated with increased risk of dementia and severe Alzheimer’s disease; a 2018 study, for example, found elevated TMAO levels in the cerebrospinal fluid of Alzheimer’s patients compared to healthy controls.
Choline and Asthma
Choline may be beneficial for people with asthma. A 2010 study, for example, found that choline supplementation modulated immune inflammation and suppressed oxidative stress in asthma patients, while a 1997 placebo-controlled study found that choline supplementation decreased asthma symptoms and increased the number of symptom-free days in a dose-dependent manner.
Didn’t know choline was this fascinating? Maybe your friends will enjoy this too!
Health Effects of Choline Deficiency
About 90% of the US population is estimated to have insufficient choline intake, and certain genetic and lifestyle factors can further increase the risk of choline insufficiency or deficiency. For example, some polyphorphisms of the PEMT gene (involved in the synthesis of phosphatidylcholine) can raise dietary choline requirements, in turn increasing the risk of choline deficiency. Defects in the choline transporter-like protein 1, a protein that allows for choline uptake into muscle, could likewise increase a person’s choline needs. High intakes of alcohol and frequent high-intensity exercise can also deplete choline levels more rapidly, increasing the risk of health problems associated with deficiency or inadequacy.
When choline deficiency occurs, it can lead to excess fat deposition in the liver (NAFLD), muscle damage, fatigue, organ dysfunction, kidney necrosis, increased DNA damage, elevated homocysteine levels, and neurological issues such as impaired brain function and memory. In pregnant women, choline deficiency can lead to neural tube defects in the developing fetus, due to the role of choline in neural tube closure.
Notably, premenopausal women are more protected from choline deficiency than other groups! The hormone estrogen induces PEMT enzyme activity, increasing the body’s synthesis of phosphatidylcholine. As a result, populations with the highest estrogen levels (premenopausal women) have higher endogenous choline production and lower dietary choline needs than groups with lower estrogen levels (men and postmenopausal women)! In fact, a 2007 randomized controlled trial found that after feeding participants choline-deficient diets for up to 42 days, only 44% of premenopausal women developed signs of organ dysfunction (including fatty liver and muscle damage), compared to 77% of male participants and 80% of postmenopausal women.
Want to know the top 500 most nutrient-dense foods?
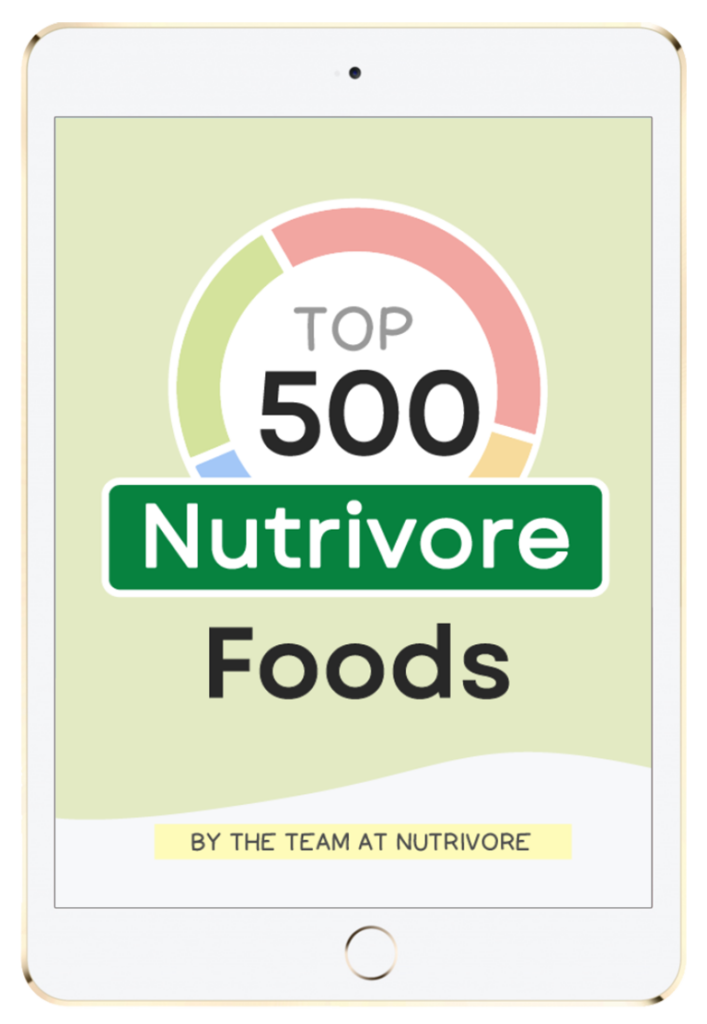
Top 500 Nutrivore Foods
The Top 500 Nutrivore Foods e-book is an amazing reference deck of the top 500 most nutrient-dense foods according to their Nutrivore Score. Think of it as the go-to resource for a super-nerd, to learn more and better understand which foods stand out, and why!
If you are looking for a quick-reference guide to help enhance your diet with nutrients, and dive into the details of your favorite foods, this book is your one-stop-shop!
Buy now for instant digital access.
Problems From Too Much Choline
Extremely high intakes of choline—typically in excess of 7,500 mg daily—have been associated with some adverse side effects. In particular, a fishy body odor (due to excess production of TMAO), low blood pressure, sweating, gastrointestinal distress, nausea, and vomiting can occur. Furthermore, some studies suggest that excessive choline intake may be linked to an increased risk of cardiovascular diseases and prostate cancer—although further research is needed to establish a clear cause-and-effect relationship. As a result of these potential toxicity issues, the tolerable upper intake level for choline is set at 3,500 mg daily.
How Much Choline Do We Need?
There’s currently no recommended dietary allowance set for choline, but adequate intake (AI) levels established by the Food and Nutrition Board are set at 550 mg daily for men and 425 mg daily for women. During pregnancy, the AI increases to 450 mg per day, and during lactation, it increases to 550 mg. However, human trials have shown that some people (especially men) develop signs of choline deficiency even when meeting the recommended AI. So, in some cases, higher intakes may be necessary to support optimal health.
0 – 6 months | |||||
6 months to < 12 months | |||||
1 yr – 3 yrs | |||||
4 yrs – 8 yrs | |||||
9 yrs – 13 yrs | |||||
14 yrs – 18 yrs | |||||
19 yrs – 50 yrs | |||||
51+ yrs | |||||
Pregnant (14 – 18 yrs) | |||||
Pregnant (19 – 30 yrs) | |||||
Pregnant (31 – 50 yrs) | |||||
Lactating (14 – 18 yrs) | |||||
Lactating (19 – 30 yrs) | |||||
Lactating (31 – 50 yrs) |
Nutrient Daily Values
Nutrition requirements and recommended nutrient intake for infants, children, adolescents, adults, mature adults, and pregnant and lactating individuals.
Best Food Sources of Choline
The following foods have high concentrations of choline, containing at least 50% DV per serving, making them our best food sources of this valuable mineral!
Easily track your servings of Nutrivore Foundational Foods!
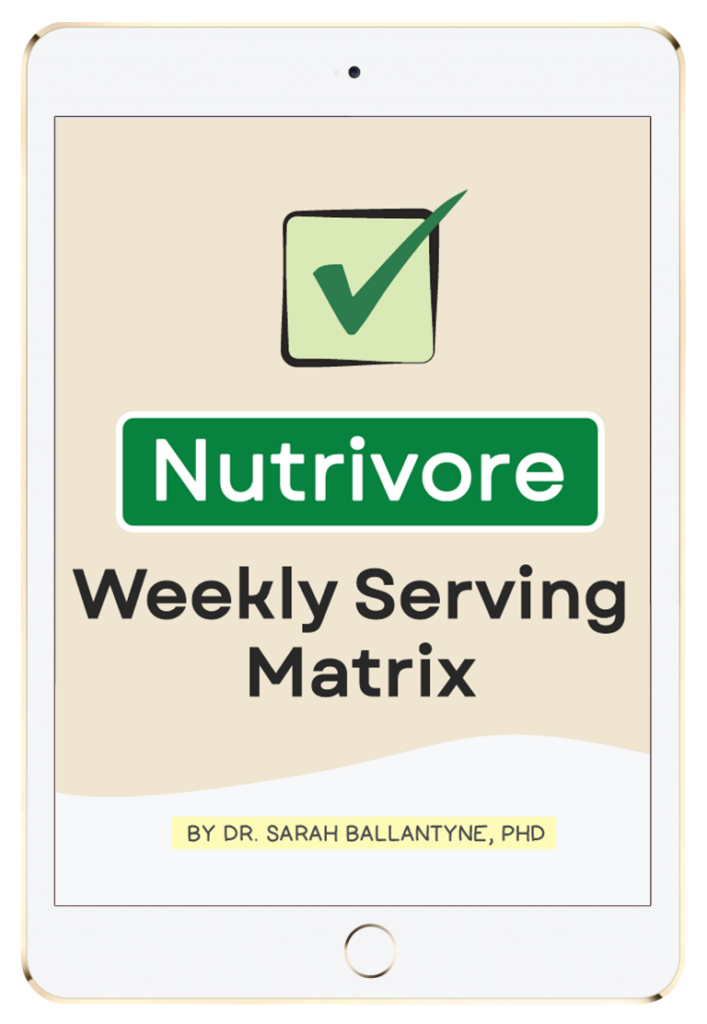
The Nutrivore Weekly Serving Matrix
The Nutrivore Weekly Serving Matrix digital resource is an easy-to-use and flexible weekly checklist designed to help you maximize nutrient-density and meet serving suggestions of Nutrivore foundational foods, all without having to weigh or measure your foods!
Includes a 22-page instructional guide and downloadable interactive guides.
Buy now for instant digital access.
Good Food Sources of Choline
The following foods are also excellent or good sources of choline, containing at least 10% DV (and up to 50% DV) per serving.
Citations
Expand to see all scientific references for this article.
References
Al Rajabi A, Castro GS, da Silva RP, Nelson RC, Thiesen A, Vannucchi H, Vine DF, Proctor SD, Field CJ, Curtis JM, Jacobs RL. Choline supplementation protects against liver damage by normalizing cholesterol metabolism in Pemt/Ldlr knockout mice fed a high-fat diet. J Nutr. 2014 Mar;144(3):252-7. doi: 10.3945/jn.113.185389.
Arumugam MK, Paal MC, Donohue TM Jr, Ganesan M, Osna NA, Kharbanda KK. Beneficial Effects of Betaine: A Comprehensive Review. Biology (Basel). 2021 May 22;10(6):456. doi: 10.3390/biology10060456.
Ashraf MA, Nookala V. Biochemistry of Platelet Activating Factor. [Updated 2023 Apr 10]. In: StatPearls [Internet]. Treasure Island (FL): StatPearls Publishing; 2023 Jan-. Available from: https://www.ncbi.nlm.nih.gov/books/NBK557392/
Böckmann KA, Franz AR, Minarski M, Shunova A, Maiwald CA, Schwarz J, Gross M, Poets CF, Bernhard W. Differential metabolism of choline supplements in adult volunteers. Eur J Nutr. 2022 Feb;61(1):219-230. doi: 10.1007/s00394-021-02637-6.
Canty DJ, Zeisel SH. Lecithin and choline in human health and disease. Nutr Rev. 1994 Oct;52(10):327-39. doi: 10.1111/j.1753-4887.1994.tb01357.x.
Caudill MA, Strupp BJ, Muscalu L, Nevins JEH, Canfield RL. Maternal choline supplementation during the third trimester of pregnancy improves infant information processing speed: a randomized, double-blind, controlled feeding study. FASEB J. 2018 Apr;32(4):2172-2180. doi: 10.1096/fj.201700692RR.
Chai C, Chen L, Deng MG, Liang Y, Liu F, Nie JQ. Dietary choline intake and non-alcoholic fatty liver disease (NAFLD) in U.S. adults: National Health and Nutrition Examination Survey (NHANES) 2017-2018. Eur J Clin Nutr. 2023 Aug 26. doi: 10.1038/s41430-023-01336-1.
Chan CWH, Law BMH, Waye MMY, Chan JYW, So WKW, Chow KM. Trimethylamine-N-oxide as One Hypothetical Link for the Relationship between Intestinal Microbiota and Cancer – Where We Are and Where Shall We Go? J Cancer. 2019 Oct 8;10(23):5874-5882. doi: 10.7150/jca.31737.
Cho CE, Aardema NDJ, Bunnell ML, Larson DP, Aguilar SS, Bergeson JR, Malysheva OV, Caudill MA, Lefevre M. Effect of Choline Forms and Gut Microbiota Composition on Trimethylamine-N-Oxide Response in Healthy Men. Nutrients. 2020 Jul 25;12(8):2220. doi: 10.3390/nu12082220.
Cox MA, Bassi C, Saunders ME, Nechanitzky R, Morgado-Palacin I, Zheng C, Mak TW. Beyond neurotransmission: acetylcholine in immunity and inflammation. J Intern Med. 2020 Feb;287(2):120-133. doi: 10.1111/joim.13006.
da Costa KA, Kozyreva OG, Song J, Galanko JA, Fischer LM, Zeisel SH. Common genetic polymorphisms affect the human requirement for the nutrient choline. FASEB J. 2006 Jul;20(9):1336-44. doi: 10.1096/fj.06-5734com.
Derbyshire E, Maes M. The Role of Choline in Neurodevelopmental Disorders-A Narrative Review Focusing on ASC, ADHD and Dyslexia. Nutrients. 2023 Jun 25;15(13):2876. doi: 10.3390/nu15132876.
DiMarco DM, Missimer A, Murillo AG, Lemos BS, Malysheva OV, Caudill MA, Blesso CN, Fernandez ML. Intake of up to 3 Eggs/Day Increases HDL Cholesterol and Plasma Choline While Plasma Trimethylamine-N-oxide is Unchanged in a Healthy Population. Lipids. 2017 Mar;52(3):255-263. doi: 10.1007/s11745-017-4230-9.
Fischer LM, da Costa KA, Kwock L, Galanko J, Zeisel SH. Dietary choline requirements of women: effects of estrogen and genetic variation. Am J Clin Nutr. 2010 Nov;92(5):1113-9. doi: 10.3945/ajcn.2010.30064. Epub 2010 Sep 22.
Fischer LM, daCosta KA, Kwock L, Stewart PW, Lu TS, Stabler SP, Allen RH, Zeisel SH. Sex and menopausal status influence human dietary requirements for the nutrient choline. Am J Clin Nutr. 2007 May;85(5):1275-85. doi: 10.1093/ajcn/85.5.1275.
Gabis LV, Ben-Hur R, Shefer S, Jokel A, Shalom DB. Improvement of Language in Children with Autism with Combined Donepezil and Choline Treatment. J Mol Neurosci. 2019 Oct;69(2):224-234. doi: 10.1007/s12031-019-01351-7.
Gaur SN, Agarwal G, Gupta SK. Use of LPC antagonist, choline, in the management of bronchial asthma. Indian J Chest Dis Allied Sci. 1997 Apr-Jun;39(2):107-13.
Gawrys-Kopczynska M, Konop M, Maksymiuk K, Kraszewska K, Derzsi L, Sozanski K, Holyst R, Pilz M, Samborowska E, Dobrowolski L, Jaworska K, Mogilnicka I, Ufnal M. TMAO, a seafood-derived molecule, produces diuresis and reduces mortality in heart failure rats. Elife. 2020 Jun 8;9:e57028. doi: 10.7554/eLife.57028.
Golzarand M, Mirmiran P, Azizi F. Association between dietary choline and betaine intake and 10.6-year cardiovascular disease in adults. Nutr J. 2022 Jan 5;21(1):1. doi: 10.1186/s12937-021-00755-9.
Guerrerio AL, Colvin RM, Schwartz AK, Molleston JP, Murray KF, Diehl A, Mohan P, Schwimmer JB, Lavine JE, Torbenson MS, Scheimann AO. Choline intake in a large cohort of patients with nonalcoholic fatty liver disease. Am J Clin Nutr. 2012 Apr;95(4):892-900. doi: 10.3945/ajcn.111.020156.
Hamlin JC, Pauly M, Melnyk S, Pavliv O, Starrett W, Crook TA, James SJ. Dietary intake and plasma levels of choline and betaine in children with autism spectrum disorders. Autism Res Treat. 2013;2013:578429. doi: 10.1155/2013/578429. Epub 2013 Dec 16.
Hammond JW, Lu SM, Gelbard HA. Platelet Activating Factor Enhances Synaptic Vesicle Exocytosis Via PKC, Elevated Intracellular Calcium, and Modulation of Synapsin 1 Dynamics and Phosphorylation. Front Cell Neurosci. 2016 Jan 8;9:505. doi: 10.3389/fncel.2015.00505. Erratum in: Front Cell Neurosci. 2016;10:113.
Han P, Bidulescu A, Barber JR, Zeisel SH, Joshu CE, Prizment AE, Vitolins MZ, Platz EA. Dietary choline and betaine intakes and risk of total and lethal prostate cancer in the Atherosclerosis Risk in Communities (ARIC) Study. Cancer Causes Control. 2019 Apr;30(4):343-354. doi: 10.1007/s10552-019-01148-4.
He GD, Liu XC, Lu AS, Feng YQ. Association of Choline Intake with Blood Pressure and Effects of Its Microbiota-Dependent Metabolite Trimethylamine-N-Oxide on Hypertension. Cardiovasc Ther. 2022 Aug 25;2022:9512401. doi: 10.1155/2022/9512401.
Huc T, Drapala A, Gawrys M, Konop M, Bielinska K, Zaorska E, Samborowska E, Wyczalkowska-Tomasik A, Pączek L, Dadlez M, Ufnal M. Chronic, low-dose TMAO treatment reduces diastolic dysfunction and heart fibrosis in hypertensive rats. Am J Physiol Heart Circ Physiol. 2018 Dec 1;315(6):H1805-H1820. doi: 10.1152/ajpheart.00536.2018.
Irvine N, England-Mason G, Field CJ, Dewey D, Aghajafari F. Prenatal Folate and Choline Levels and Brain and Cognitive Development in Children: A Critical Narrative Review. Nutrients. 2022 Jan 15;14(2):364. doi: 10.3390/nu14020364.
Jennings L, Basiri R. Amino Acids, B Vitamins, and Choline May Independently and Collaboratively Influence the Incidence and Core Symptoms of Autism Spectrum Disorder. Nutrients. 2022 Jul 14;14(14):2896. doi: 10.3390/nu14142896.
Johansson M, Van Guelpen B, Vollset SE, Hultdin J, Bergh A, Key T, Midttun O, Hallmans G, Ueland PM, Stattin P. One-carbon metabolism and prostate cancer risk: prospective investigation of seven circulating B vitamins and metabolites. Cancer Epidemiol Biomarkers Prev. 2009 May;18(5):1538-43. doi: 10.1158/1055-9965.EPI-08-1193.
Judd JM, Jasbi P, Winslow W, Serrano GE, Beach TG, Klein-Seetharaman J, Velazquez R. Inflammation and the pathological progression of Alzheimer’s disease are associated with low circulating choline levels. Acta Neuropathol. 2023 Oct;146(4):565-583. doi: 10.1007/s00401-023-02616-7.
Korsmo HW, Jiang X, Caudill MA. Choline: Exploring the Growing Science on Its Benefits for Moms and Babies. Nutrients. 2019 Aug 7;11(8):1823. doi: 10.3390/nu11081823.
Li Z, Vance DE. Phosphatidylcholine and choline homeostasis. J Lipid Res. 2008 Jun;49(6):1187-94. doi: 10.1194/jlr.R700019-JLR200.
Liu D, Bu D, Li H, Wang Q, Ding X, Fang X. Intestinal metabolites and the risk of autistic spectrum disorder: A two-sample Mendelian randomization study. Front Psychiatry. 2023 Jan 12;13:1034214. doi: 10.3389/fpsyt.2022.1034214.
Liu L, Qiao S, Zhuang L, Xu S, Chen L, Lai Q, Wang W. Choline Intake Correlates with Cognitive Performance among Elder Adults in the United States. Behav Neurol. 2021 Oct 29;2021:2962245. doi: 10.1155/2021/2962245.
Lu MS, Fang YJ, Pan ZZ, Zhong X, Zheng MC, Chen YM, Zhang CX. Choline and betaine intake and colorectal cancer risk in Chinese population: a case-control study. PLoS One. 2015 Mar 18;10(3):e0118661. doi: 10.1371/journal.pone.0118661.
Mashimo M, Moriwaki Y, Misawa H, Kawashima K, Fujii T. Regulation of Immune Functions by Non-Neuronal Acetylcholine (ACh) via Muscarinic and Nicotinic ACh Receptors. Int J Mol Sci. 2021 Jun 24;22(13):6818. doi: 10.3390/ijms22136818.
Mazidi M, Katsiki N, Mikhailidis DP, Banach M. Adiposity May Moderate the Link Between Choline Intake and Non-alcoholic Fatty Liver Disease. J Am Coll Nutr. 2019 Sep-Oct;38(7):633-639. doi: 10.1080/07315724.2018.1507011.
Mazidi M, Katsiki N, Mikhailidis DP, Banach M. Dietary choline is positively related to overall and cause-specific mortality: results from individuals of the National Health and Nutrition Examination Survey and pooling prospective data. Br J Nutr. 2019 Dec 14;122(11):1262-1270. doi: 10.1017/S0007114519001065.
Mehta AK, Singh BP, Arora N, Gaur SN. Choline attenuates immune inflammation and suppresses oxidative stress in patients with asthma. Immunobiology. 2010 Jul;215(7):527-34. doi: 10.1016/j.imbio.2009.09.004.
Meyer KA, Shea JW. Dietary Choline and Betaine and Risk of CVD: A Systematic Review and Meta-Analysis of Prospective Studies. Nutrients. 2017 Jul 7;9(7):711. doi: 10.3390/nu9070711.
Millard HR, Musani SK, Dibaba DT, Talegawkar SA, Taylor HA, Tucker KL, Bidulescu A. Dietary choline and betaine; associations with subclinical markers of cardiovascular disease risk and incidence of CVD, coronary heart disease and stroke: the Jackson Heart Study. Eur J Nutr. 2018 Feb;57(1):51-60. doi: 10.1007/s00394-016-1296-8.
Mondul AM, Moore SC, Weinstein SJ, Karoly ED, Sampson JN, Albanes D. Metabolomic analysis of prostate cancer risk in a prospective cohort: The alpha-tocolpherol, beta-carotene cancer prevention (ATBC) study. Int J Cancer. 2015 Nov 1;137(9):2124-32. doi: 10.1002/ijc.29576.
Nagata C, Wada K, Tamura T, Konishi K, Kawachi T, Tsuji M, Nakamura K. Choline and Betaine Intakes Are Not Associated with Cardiovascular Disease Mortality Risk in Japanese Men and Women. J Nutr. 2015 Aug;145(8):1787-92. doi: 10.3945/jn.114.209296.
Nurk E, Refsum H, Bjelland I, Drevon CA, Tell GS, Ueland PM, Vollset SE, Engedal K, Nygaard HA, Smith DA. Plasma free choline, betaine and cognitive performance: the Hordaland Health Study. Br J Nutr. 2013 Feb 14;109(3):511-9. doi: 10.1017/S0007114512001249.
Obeid R, Derbyshire E, Schön C. Association between Maternal Choline, Fetal Brain Development, and Child Neurocognition: Systematic Review and Meta-Analysis of Human Studies. Adv Nutr. 2022 Dec 22;13(6):2445-2457. doi: 10.1093/advances/nmac082.
Poly C, Massaro JM, Seshadri S, Wolf PA, Cho E, Krall E, Jacques PF, Au R. The relation of dietary choline to cognitive performance and white-matter hyperintensity in the Framingham Offspring Cohort. Am J Clin Nutr. 2011 Dec;94(6):1584-91. doi: 10.3945/ajcn.110.008938.
Richman EL, Kenfield SA, Stampfer MJ, Giovannucci EL, Zeisel SH, Willett WC, Chan JM. Choline intake and risk of lethal prostate cancer: incidence and survival. Am J Clin Nutr. 2012 Oct;96(4):855-63. doi: 10.3945/ajcn.112.039784.
Ridgway ND. The role of phosphatidylcholine and choline metabolites to cell proliferation and survival. Crit Rev Biochem Mol Biol. 2013 Jan-Feb;48(1):20-38. doi: 10.3109/10409238.2012.735643.
Rossi M, Khalifeh M, Fiori F, Parpinel M, Serraino D, Pelucchi C, Negri E, Giacosa A, Crispo A, Collatuzzo G, Hannun Y, Luberto C, La Vecchia C, Boffetta P. Dietary choline and sphingomyelin choline moiety intake and risk of colorectal cancer: a case-control study. Eur J Clin Nutr. 2023 Sep;77(9):905-910. doi: 10.1038/s41430-023-01298-4.
Shaw GM, Carmichael SL, Yang W, Selvin S, Schaffer DM. Periconceptional dietary intake of choline and betaine and neural tube defects in offspring. Am J Epidemiol. 2004 Jul 15;160(2):102-9. doi: 10.1093/aje/kwh187.
Shaw GM, Finnell RH, Blom HJ, Carmichael SL, Vollset SE, Yang W, Ueland PM. Choline and risk of neural tube defects in a folate-fortified population. Epidemiology. 2009 Sep;20(5):714-9. doi: 10.1097/EDE.0b013e3181ac9fe7.
Song M, Xu BP, Liang Q, Wei Y, Song Y, Chen P, Zhou Z, Zhang N, He Q, Liu L, Liu T, Zhang K, Hu C, Wang B, Xu X, Shi H. Association of serum choline levels and all-cause mortality risk in adults with hypertension: a nested case-control study. Nutr Metab (Lond). 2021 Dec 20;18(1):108. doi: 10.1186/s12986-021-00637-1.
Sun S, Li X, Ren A, Du M, Du H, Shu Y, Zhu L, Wang W. Choline and betaine consumption lowers cancer risk: a meta-analysis of epidemiologic studies. Sci Rep. 2016 Oct 19;6:35547. doi: 10.1038/srep35547.
Tang WH, Wang Z, Levison BS, Koeth RA, Britt EB, Fu X, Wu Y, Hazen SL. Intestinal microbial metabolism of phosphatidylcholine and cardiovascular risk. N Engl J Med. 2013 Apr 25;368(17):1575-84. doi: 10.1056/NEJMoa1109400.
Ueland PM. Choline and betaine in health and disease. J Inherit Metab Dis. 2011 Feb;34(1):3-15. doi: 10.1007/s10545-010-9088-4.
Ufnal M, Nowiński A. Is increased plasma TMAO a compensatory response to hydrostatic and osmotic stress in cardiovascular diseases? Med Hypotheses. 2019 Sep;130:109271. doi: 10.1016/j.mehy.2019.109271.
Van Puyvelde H, Dimou N, Katsikari A, Indave Ruiz BI, Godderis L, Huybrechts I, De Bacquer D. The association between dietary intakes of methionine, choline and betaine and breast cancer risk: A systematic review and meta-analysis. Cancer Epidemiol. 2023 Apr;83:102322. doi: 10.1016/j.canep.2023.102322.
Velasquez MT, Ramezani A, Manal A, Raj DS. Trimethylamine N-Oxide: The Good, the Bad and the Unknown. Toxins (Basel). 2016 Nov 8;8(11):326. doi: 10.3390/toxins8110326.
Villamor E, Rifas-Shiman SL, Gillman MW, Oken E. Maternal intake of methyl-donor nutrients and child cognition at 3 years of age. Paediatr Perinat Epidemiol. 2012 Jul;26(4):328-35. doi: 10.1111/j.1365-3016.2012.01264.x.
Vogt NM, Romano KA, Darst BF, Engelman CD, Johnson SC, Carlsson CM, Asthana S, Blennow K, Zetterberg H, Bendlin BB, Rey FE. The gut microbiota-derived metabolite trimethylamine N-oxide is elevated in Alzheimer’s disease. Alzheimers Res Ther. 2018 Dec 22;10(1):124. doi: 10.1186/s13195-018-0451-2.
Wallace TC, Fulgoni VL 3rd. Assessment of Total Choline Intakes in the United States. J Am Coll Nutr. 2016;35(2):108-12. doi: 10.1080/07315724.2015.1080127.
Wang X, Li X, Dong Y. Vitamin D Decreases Plasma Trimethylamine-N-oxide Level in Mice by Regulating Gut Microbiota. Biomed Res Int. 2020 Oct 5;2020:9896743. doi: 10.1155/2020/9896743.
Wang Z, Tang WH, Buffa JA, Fu X, Britt EB, Koeth RA, Levison BS, Fan Y, Wu Y, Hazen SL. Prognostic value of choline and betaine depends on intestinal microbiota-generated metabolite trimethylamine-N-oxide. Eur Heart J. 2014 Apr;35(14):904-10. doi: 10.1093/eurheartj/ehu002.
Warton FL, Molteno CD, Warton CMR, Wintermark P, Lindinger NM, Dodge NC, Zöllei L, van der Kouwe AJW, Carter RC, Jacobson JL, Jacobson SW, Meintjes EM. Maternal choline supplementation mitigates alcohol exposure effects on neonatal brain volumes. Alcohol Clin Exp Res. 2021 Sep;45(9):1762-1774. doi: 10.1111/acer.14672.
Wilcox J, Skye SM, Graham B, Zabell A, Li XS, Li L, Shelkay S, Fu X, Neale S, O’Laughlin C, Peterson K, Hazen SL, Tang WHW. Dietary Choline Supplements, but Not Eggs, Raise Fasting TMAO Levels in Participants with Normal Renal Function: A Randomized Clinical Trial. Am J Med. 2021 Sep;134(9):1160-1169.e3. doi: 10.1016/j.amjmed.2021.03.016.
Yang JJ, Lipworth LP, Shu XO, Blot WJ, Xiang YB, Steinwandel MD, Li H, Gao YT, Zheng W, Yu D. Associations of choline-related nutrients with cardiometabolic and all-cause mortality: results from 3 prospective cohort studies of blacks, whites, and Chinese. Am J Clin Nutr. 2020 Mar 1;111(3):644-656. doi: 10.1093/ajcn/nqz318.
Yang Q, Han H, Sun Z, Liu L, Zheng X, Meng Z, Tao N, Liu J. Association of choline and betaine with the risk of cardiovascular disease and all-cause mortality: Meta-analysis. Eur J Clin Invest. 2023 Oct;53(10):e14041. doi: 10.1111/eci.14041.
Yang Y, Yang B, Li X, Xue L, Liu B, Liang Y, Zhao Z, Luo Q, Liu Z, Zeng Q, Xiong C. Higher circulating Trimethylamine N-oxide levels are associated with worse severity and prognosis in pulmonary hypertension: a cohort study. Respir Res. 2022 Dec 14;23(1):344. doi: 10.1186/s12931-022-02282-5.
Ylilauri MPT, Voutilainen S, Lönnroos E, Virtanen HEK, Tuomainen TP, Salonen JT, Virtanen JK. Associations of dietary choline intake with risk of incident dementia and with cognitive performance: the Kuopio Ischaemic Heart Disease Risk Factor Study. Am J Clin Nutr. 2019 Dec 1;110(6):1416-1423. doi: 10.1093/ajcn/nqz148.
Yu D, Shu XO, Xiang YB, Li H, Yang G, Gao YT, Zheng W, Zhang X. Higher dietary choline intake is associated with lower risk of nonalcoholic fatty liver in normal-weight Chinese women. J Nutr. 2014 Dec;144(12):2034-40. doi: 10.3945/jn.114.197533.
Yuan J, Liu X, Liu C, Ang AF, Massaro J, Devine SA, Auerbach SH, Blusztajn JK, Au R, Jacques PF. Is dietary choline intake related to dementia and Alzheimer’s disease risks? Results from the Framingham Heart Study. Am J Clin Nutr. 2022 Nov;116(5):1201-1207. doi: 10.1093/ajcn/nqac193.
Zeisel SH, da Costa KA. Choline: an essential nutrient for public health. Nutr Rev. 2009 Nov;67(11):615-23. doi: 10.1111/j.1753-4887.2009.00246.x.
Zeisel SH. A brief history of choline. Ann Nutr Metab. 2012;61(3):254-8. doi: 10.1159/000343120. Epub 2012 Nov 26.
Zhang CX, Pan MX, Li B, Wang L, Mo XF, Chen YM, Lin FY, Ho SC. Choline and betaine intake is inversely associated with breast cancer risk: a two-stage case-control study in China. Cancer Sci. 2013 Feb;104(2):250-8. doi: 10.1111/cas.12064.
Zheng Y, Li Y, Rimm EB, Hu FB, Albert CM, Rexrode KM, Manson JE, Qi L. Dietary phosphatidylcholine and risk of all-cause and cardiovascular-specific mortality among US women and men. Am J Clin Nutr. 2016 Jul;104(1):173-80. doi: 10.3945/ajcn.116.131771.
Zhou R, Yang M, Yue C, Shi Y, Tan Y, Zha L, Zhang J, Chen S. Association between Dietary Choline Intake and Cardiovascular Diseases: National Health and Nutrition Examination Survey 2011-2016. Nutrients. 2023 Sep 18;15(18):4036. doi: 10.3390/nu15184036.
Zhou RF, Chen XL, Zhou ZG, Zhang YJ, Lan QY, Liao GC, Chen YM, Zhu HL. Higher dietary intakes of choline and betaine are associated with a lower risk of primary liver cancer: a case-control study. Sci Rep. 2017 Apr 6;7(1):679. doi: 10.1038/s41598-017-00773-w.