Key Takeaways (expand)
- Methionine is one of the nine essential amino acids that we must consume from our diets, and one of the 20 amino acids that can help build proteins.
- Methionine is also one of only a handful of sulfur-containing amino acids—the others being cysteine, taurine, and homocysteine.
- Due to its sulfur content, methionine makes a significant contribution to the body’s available pool of sulfur (which is then needed for numerous biological functions).
- Methionine serves as a precursor to a number of other important molecules, including the methyl donor S-adenosyl methionine (SAMe), phosphatidylcholine, the antioxidant glutathione, and the amino acids homocysteine, cysteine, carnitine, taurine, and creatine.
- Through its precursor role, methionine contributes to tissue building and repair, immune system function, the breakdown of neurotransmitters, hormone regulation, detoxification, metabolism, digestion, and antioxidant defense.
- Some studies, although mostly observational, suggest a protective effect of dietary methionine on liver cancer, pancreatic cancer, breast cancer, and colorectal cancer.
- Excess methionine has been linked to increased risk of cardiovascular disease, non-alcoholic fatty liver disease, and certain cancers, especially in animal models—although the evidence is less compelling in humans.
- Some preliminary evidence suggests high intakes of methionine could worsen schizophrenia symptoms and induce detrimental changes in the gut microbiome.
- Good sources of methionine include eggs, chicken, beef, pork, fish, Brazil nuts, sesame seeds, hempseeds, chia seeds, and soybeans.
Table of Contents[Hide][Show]
Methionine is one of the nine essential amino acids, and one of only two proteinogenic sulfur-containing amino acids (the other being cysteine). It was first isolated in 1921 by the American biochemist John Howard Mueller.
Along with being used for protein biosynthesis, methionine serves as a precursor for other amino acids, glutathione (AKA the “master antioxidant!”), the methyl donor SAMe, and other sulfur-containing compounds. This gives it an important role in numerous metabolic processes, immune function, and digestion!
Good sources of methionine include eggs, chicken, beef, pork, fish, Brazil nuts, sesame seeds, hempseeds, chia seeds, and soybeans.
The Biological Roles of Methionine
Broadly, amino acids are molecules with the molecular formula of R-CH(NH2)-COOH-NH2, where -NH2 is the basic amino group, COOH is an acidic carboxyl group, and R represents a molecular unit called a side chain. That side chain is unique for each amino acid, and its chemical properties create different classes of amino acids: nonpolar and neutral, polar and neutral, polar and acidic, and polar and basic.
Although hundreds of amino acids exist, only 20 of them are used for what amino acids are arguably most known for: forming the building blocks of proteins. Proteins are not only an essential macronutrient in the human diet; they’re molecules that perform most of the various functions of life. In addition to being major structural components of cells and tissues, proteins have incredibly diverse roles that range from driving chemical reactions (e.g., enzymes) to signaling (e.g., some types of hormones) to transporting and storing nutrients. Proteins are synthesized within cells through a two-phase process of transcription and translation, during which amino acids get linked together to form long chains (spanning anywhere from 20 to over 2,000 amino acids in length!).
So, while all proteins are made of amino acids, not all amino acids are used for making proteins! We use the term proteinogenic amino acids to refer specifically to the amino acids that get encoded into our DNA and incorporated into proteins. Meanwhile, non-proteinogenic amino acids do neither of these things (although they still have some very important biological roles!).
Amino acids can be further classified based on whether we can create them in our bodies, or need them from our diet. Essential amino acids are amino acids that can only be obtained from foods; these include histidine, isoleucine, leucine, lysine, methionine, phenylalanine, threonine, tryptophan, and valine. Non-essential amino acids are amino acids our bodies can synthesize metabolically from other molecules; these include alanine, arginine, asparagine, aspartic acid, cysteine, glutamic acid, glutamine, glycine, proline, serine, and tyrosine. And, some amino acids are conditionally essential, meaning our bodies can normally make them, but some circumstances (like illness or stress) can limit their synthesis and create a dietary requirement. These include arginine, cysteine, glutamine, tyrosine, glycine, ornithine, proline, and serine.
As an essential amino acid, methionine has to come from the foods we eat; we can’t produce it on our own. And, as a proteinogenic amino acid, methionine is used by the body for protein synthesis!
Methionine is also one of only a handful of sulfur-containing amino acids—the others being cysteine, taurine, and homocysteine (though the latter two aren’t incorporated into proteins). Due to its sulfur content, methionine makes a significant contribution to the body’s available pool of sulfur, which is needed for electron transport, cell structure, metabolism, synthesizing and repairing DNA, supporting skin and tendon health, and more. And because sulfur is more electronegative than oxygen, its presence in methionine influences many of its molecular properties, including how hydrophobic methionine is!
Within the body (and especially within the liver), methionine is broken down and regenerated in a series of reactions called the methionine cycle. This cycle results in the production of a number of important molecules with a range of biological functions. First, methionine is converted to the universal methyl donor S-adenosyl methionine (SAMe) through the activity of an enzyme called methionine adenosyltransferase; SAMe then gets converted to S-adenosyl homocysteine (SAH), and then to the amino acid homocysteine—which can then be recycled to form methionine again! What’s more, these methionine metabolites can go on to form other critical molecules: for example, homocysteine can be converted into cystathione and then to the amino acid cysteine, which can then be used to synthesize the critical antioxidant glutathione. Methionine also serves as a precursor to phospholipids (such as phosphatidylcholine) and additional non-essential amino acids (including carnitine, taurine, and creatine).
Through its precursor role, methionine ultimately assists in the functions of the various molecules it helps form. By helping produce glutathione, for example, methionine supports tissue building and repair, antioxidant function, and immune system function. The conversion of methionine into SAMe gives methionine a role in modifying DNA, breaking down neurotransmitters, maintaining cell membranes, regulating hormones, and activating various chemicals. The production of cysteine from methionine contributes to cysteine’s powerful antioxidant properties and detoxification abilities. And by helping produce taurine, methionine supports energy production, metabolism, digestion, nerve growth and nervous system functioning, the processing of bile acids, and fluid balance!
Nutrivore Is a Game-Changer—These 5 Free Guides Show You Why
Sign up for the free weekly Nutrivore Newsletter and get 5 high-value downloads—delivered straight to your inbox—that make healthy eating simple and sustainable.
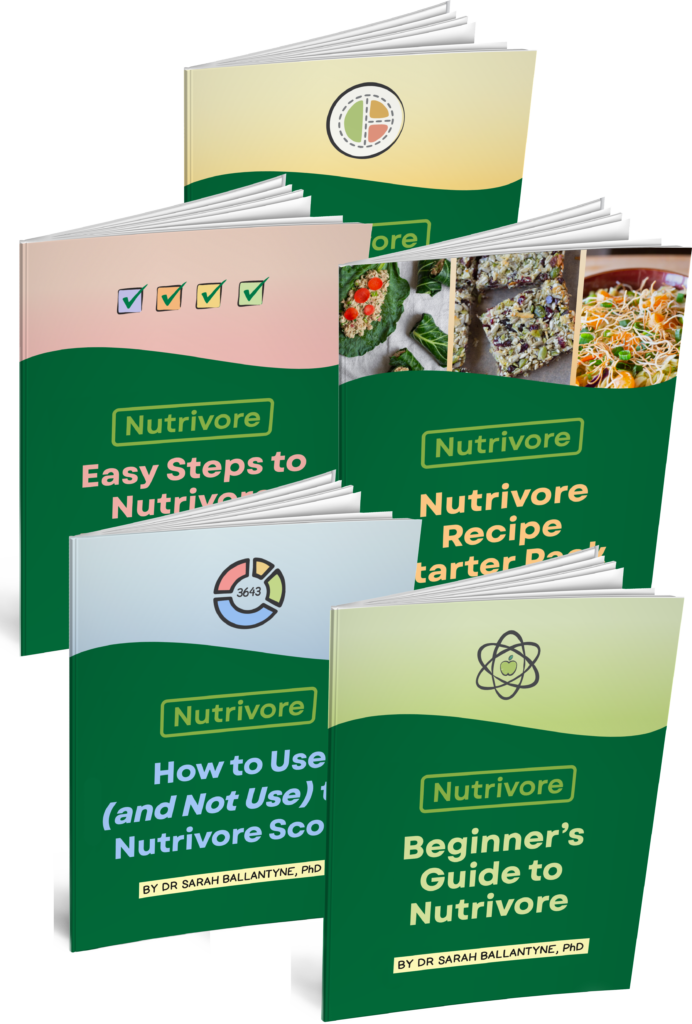
Methionine in Health and Disease
Most research on methionine has focused on the potential longevity and anti-cancer benefits that come from restricting its intake, especially in animal models. But, some evidence (albeit mostly observational) suggests methionine could play a protective role in certain cancers.
Methionine and Liver Cancer
Compared to other cancer types, methionine may have a uniquely protective role in liver cancer—possibly due to the liver being the site of both methionine synthesis and degradation. In a 2020 experiment using liver cancer cells, for example, methionine supplementation reduced the cancer cells’ growth rate and triggered some cancer-protective changes in metabolic pathways. Another in vitro experiment from 2017found that supplementation with SAMe, formed from methionine, was able to block liver cancer cell growth and inhibit liver cancer cell invasion. A mouse study from 2017 likewise found that short-term supplementation with SAMe significantly decreased the number of tumor nodules and proliferating liver cells, while also increasing the expression of tumor suppressor proteins. (Interestingly, these effects reversed with longer-term SAMe supplementation—suggesting that different mechanisms come into play after an initial induction of cell cycle arrest!)
All that being said, much more research is need in humans in order to understand whether methionine (or molecules formed from methionine) are protective against liver cancer in living humans.
Methionine and Pancreatic Cancer
A 2007 prospective study, following nearly 82,000 Swedish adults for an average of seven years, found that methionine intake was significantly inversely associated with risk of pancreatic cancer. Men in the highest versus lowest quartile of methionine intake had a 68% lower risk, while women had a 41% lower risk (for all participants combined, the risk was 56% lower). Although this study can’t prove cause and effect, findings from in vitro and animal studies support the idea that nutrients involved in methyl group metabolism (including methionine) could help reduce pancreatic carcinogenesis, due to their protective influence on toxic damage and cellular differentiation in the pancreas. More research is needed in humans!
Methionine and Breast Cancer
A protective effect of methionine intake on breast cancer has been found in some observational studies. A 2013 meta-analysis found that among post-menopausal (but not pre-menopausal) women, risk of breast cancer dropped 4% for every 1 g per day increase of methionine intake. A 2013 observational study found that methionine intake appeared protective of estrogen receptor positive breast cancer, with women in the highest quartile of intake having a 17% lower risk (29% lower risk for Hispanic women, specifically). And, some mechanistic studies have shown that methionine is cytotoxic to human breast cancer cells, leading to lower proliferation and growth—largely by reducing the expression of a key cell cycle regulatory protein called p53. Controlled studies are needed in humans to further explore these findings!
Methionine and Colorectal Cancer
A 2013 meta-analysis of eight prospective studies, encompassing over 431,000 participants, found a significant inverse association between methionine intake and colorectal cancer. Specifically, people with the highest versus lowest intake of methionine had an 11% lower risk of developing colorectal cancer, a 23% lower risk of developing colon cancer, and a 12% lower risk of developing rectal cancer. A stratified analysis of the data found that men had a particularly significant drop in colorectal cancer risk with higher methionine intake—25%, to be exact!
Likewise, a cohort study from 2008, tracking nearly 121,000 people, found that men in the highest versus lowest quintile of methionine intake had a 43% lower risk of proximal colon cancer, while women in the highest versus lowest quintile of intake had a 55% lower risk of rectal cancer. And, a 2014 study of people with Lynch syndrome (a type of inherited cancer syndrome) found that for people carrying the MTHFR 677TT allele (which impacts methionine metabolism), a low methionine intake was associated with a significantly higher risk of developing colorectal tumors.
Although these observational studies can’t confirm causation, some animal studies have also supported a protective effect of methionine and its metabolites on colorectal cancer. For example, a mouse study from 2012 found that supplementation with SAMe inhibited several pathways involved in colon carcinogenesis, inducing apoptosis and reducing colon cancer tumor load by 40%. Additional animal research has likewise shown that methionine can help reduce inflammation-induced colon cancer.
On the whole, some plausible mechanisms exist for a protective role of methionine on colorectal cancer risk, but more research is needed!
Didn’t know methionine was this interesting? Maybe your friends will enjoy this too!
Health Effects of Methionine Deficiency
Long-term methionine deficiency can lead to stunted growth, reproductive issues, fertility loss, hair loss, liver dysfunction, bone-related disorders, and elevated homocysteine levels (hyperhomocysteinemia).
Everything You Need to Jump into Nutrivore TODAY!
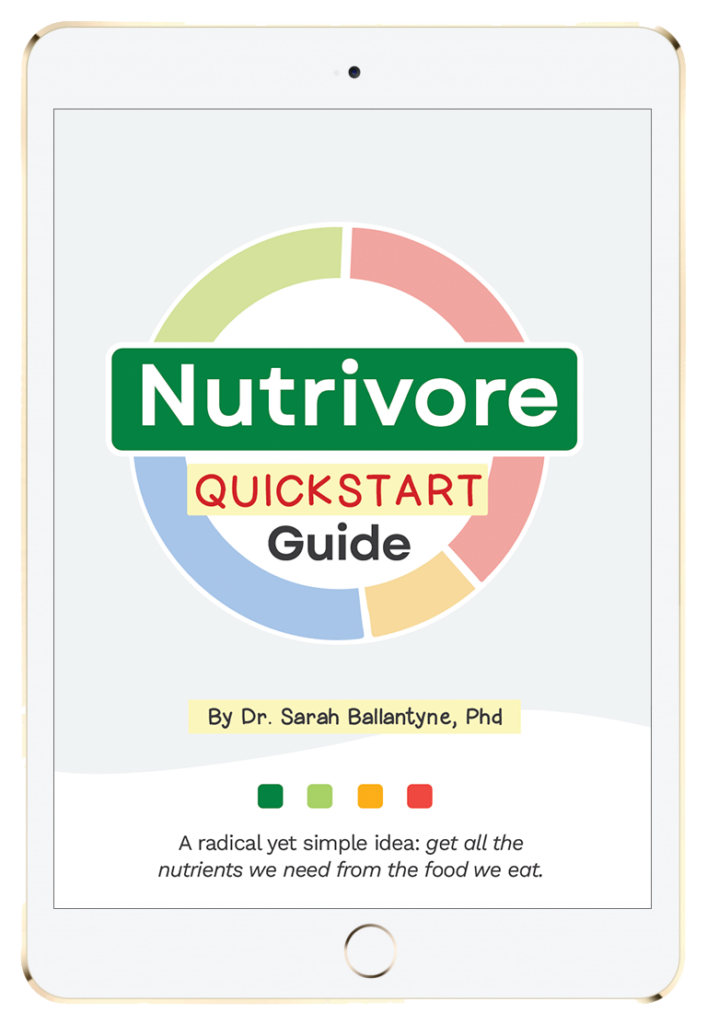
Nutrivore Quickstart Guide
The Nutrivore Quickstart Guide e-book explains why and how to eat a Nutrivore diet, introduces the Nutrivore Score, gives a comprehensive tour of the full range of essential and important nutrients!
Plus, you’ll find the Top 100 Nutrivore Score Foods, analysis of food groups, practical tips to increase the nutrient density of your diet, and look-up tables for the Nutrivore Score of over 700 foods.
Buy now for instant digital access.
Problems From Too Much Methionine
Although consuming methionine is essential for human health, high intakes have been implicated in some health issues and chronic diseases.
For one, methionine is needed for the growth and metabolism of cancer cells, giving excess dietary methionine a potential role in cancer growth. In contrast to healthy cells, cancer cells have metabolic defects that make them uniquely dependent on the presence of methionine—a phenomenon known as the “Hoffman effect.” A variety of animal and in vitro experiments have shown that restricting dietary methionine helps inhibit the growth of various cancer types, including melanoma, prostate cancer, and sarcoma. And in animal models, methionine restriction has been shown to enhance the effectiveness of chemotherapy and radiation therapy.
However, while many studies suggest methionine restriction could reduce cancer growth (at least in animal models), relatively fewer studies have linked excess methionine to increased cancer growth. One case-control study from 2013 did find that among men with at least one copy of the MTHFR A1298C allele (which impairs the activity of an enzyme involved in maintaining methionine and homocysteine homeostasis), those with a high dietary methionine intake were significantly more likely to develop prostate cancer—a 670% increased risk! However, the association wasn’t present for non-carriers of this allele. Clearly, this is an area where much more research is still needed, especially where diet and genetics might intersect.
Excess methionine has also been linked to a potential increase in cardiovascular disease risk. This may be due in part to its conversion to the non-essential amino acid homocysteine, which itself has been implicated in cardiovascular injury. For example, a number of case-control and epidemiological studies have linked elevated homocysteine levels with higher risk of heart disease, atherosclerosis, arterial damage, and stroke. Mechanistically, elevated homocysteine has been shown to harm the endothelial layer, promote inflammation, and promote oxidative stress—all potentially contributing cardiovascular disease. That being said, studies of methionine supplementation in humans have shown that it typically takes very high intakes (about five times the amount usually obtained from diet), and/or the presence of nutrient deficiencies, in order for methionine to cause appreciable elevations in homocysteine.
Animal experiments have shown that independent of changes in homocysteine levels, methionine supplementation itself can induce changes associated with cardiovascular disease development. However, the results of randomized controlled trials haven’t shown a compelling effect of methionine on cardiovascular risk factors in humans. So, in all, the detrimental potential of methionine on the cardiovascular system is still under debate!
Some research suggests that high dietary methionine intake could contribute to non-alcoholic fatty liver disease—specifically by decreasing the production of hydrogen sulfide, which plays a protective role in fatty liver pathology. For example, a 2022 mouse study found that compared to a normal diet, a high-methionine diet reduced the expression of enzymes needed for the production of hydrogen sulfide, triggering increased inflammation and fat buildup in the liver. A methionine-restricted diet was able to then reverse these effects! And, a 2022 cross-sectional study found significant associations between methionine metabolites in the blood and the prevalence of non-alcoholic fatty liver disease. People in the highest versus lowest quartiles of serum S-adenosylhomocysteine levels and homocysteine levels, for example, were at a 65% and 63% higher risk (respectively) of having fatty liver. Greater levels of these methionine metabolites were also correlated with greater severity of the disease.
There’s also evidence that excess methionine could contribute to or worsen some psychiatric disorders, such as schizophrenia. Early studies from the 1960s experimented with high-dose methionine supplements for schizophrenic patients, and found that methionine actually worsened schizophrenia symptoms. And, animal experiments have used methionine supplementation to induce schizophrenia-like symptoms. Researchers hypothesize this could be due to methionine-induced alterations in DNA methylation at specific gene regions related to schizophrenia, particularly those affecting the neurotransmitter GABA.
Although the research is preliminary, methionine has shown potential to alter the gut microbiome (and its metabolites) in ways that negatively affect cognitive health. A mouse study from 2022 found that feeding the animals a high-methionine diet, compared to a regular or low-methionine diet, led to a decreased abundance of short-chain fatty acid producing bacteria such as Roseburia, Blautia, Faecalibaculum, and Bifidobacterium, as well as serotonin-producing bacteria such as Turicibacter; meanwhile, there was a significant increase in pro-inflammatory bacteria such as Escherichia-Shigella. These shifts in microbiome composition were linked to oxidative stress and inflammation in the mouse brain, and also seemed to downregulate the expression of memory-related genes. Interestingly, a low-methionine diet was able to reverse all of these results!
A number of other rodent studies have supported a role of methionine restriction for improving or protecting gut health (and in some cases, subsequently cognitive health), especially in older animals or those fed high-fat diets. However, most of these experiments compared normal methionine intake to deliberately restricted methionine intake, making it unclear whether excess methionine would worsen gut health relative to normal dietary intakes.
Some evidence suggests that reducing methionine levels in the diet could have important metabolic benefits. Across a variety of animal studies and cell culture studies, methionine restriction has been shown to decrease inflammation, adiposity (body fat), and oxidative stress, while improving insulin sensitivity and extending lifespan. However, this research hasn’t been compellingly replicated in humans, and doesn’t necessarily mean that excess methionine would exacerbate these issues!
How Much Methionine Do We Need?
For adults aged 19 and older, the recommended dietary allowance (RDA) for methionine is 14 mg daily per kg of body weight (or 6.4 mg per lb). During times of physical trauma or illness, however, methionine needs may be higher!
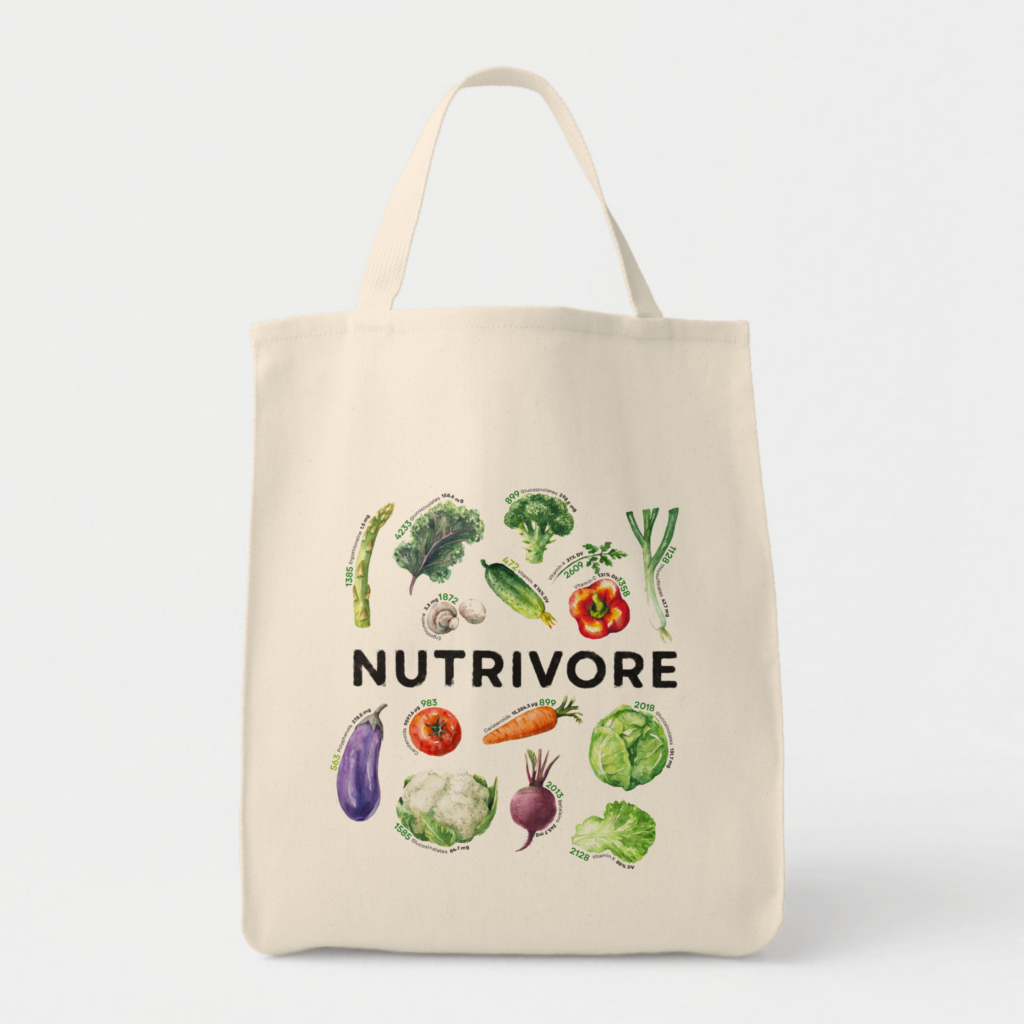
Merch and Household Goods
Check out our collection of practical and nerdtastic T-shirts, totes, and more!
Citations
Expand to see all scientific references for this article.
Aissa AF, Amaral CLD, Venancio VP, Machado CDS, Hernandes LC, Santos PWDS, Curi R, Bianchi MLP, Antunes LMG. Methionine-supplemented diet affects the expression of cardiovascular disease-related genes and increases inflammatory cytokines in mice heart and liver. J Toxicol Environ Health A. 2017;80(19-21):1116-1128. doi: 10.1080/15287394.2017.1357366.
Aissa AF, Gomes TD, Almeida MR, Hernandes LC, Darin JD, Bianchi ML, Antunes LM. Methionine concentration in the diet has a tissue-specific effect on chromosomal stability in female mice. Food Chem Toxicol. 2013 Dec;62:456-62. doi: 10.1016/j.fct.2013.09.004.
Bassila C, Ghemrawi R, Flayac J, Froese DS, Baumgartner MR, Guéant JL, Coelho D. Methionine synthase and methionine synthase reductase interact with MMACHC and with MMADHC. Biochim Biophys Acta Mol Basis Dis. 2017 Jan;1863(1):103-112. doi: 10.1016/j.bbadis.2016.10.016.
Benavides MA, Bosland MC, da Silva CP, Gomes Sares CT, de Oliveira AM, Kemp R, dos Reis RB, Martins VR, Sampaio SV, Bland KI, Grizzle WE, dos Santos JS. L-Methionine inhibits growth of human pancreatic cancer cells. Anticancer Drugs. 2014 Feb;25(2):200-3. doi: 10.1097/CAD.0000000000000038.
Brosnan JT, Brosnan ME. The sulfur-containing amino acids: an overview. J Nutr. 2006 Jun;136(6 Suppl):1636S-1640S. doi: 10.1093/jn/136.6.1636S.
Cavuoto P, Fenech MF. A review of methionine dependency and the role of methionine restriction in cancer growth control and life-span extension. Cancer Treat Rev. 2012 Oct;38(6):726-36. doi: 10.1016/j.ctrv.2012.01.004.
Chaturvedi S, Hoffman RM, Bertino JR. Exploiting methionine restriction for cancer treatment. Biochem Pharmacol. 2018 Aug;154:170-173. doi: 10.1016/j.bcp.2018.05.003.
Chrysant SG, Chrysant GS. The current status of homocysteine as a risk factor for cardiovascular disease: a mini review. Expert Rev Cardiovasc Ther. 2018 Aug;16(8):559-565. doi: 10.1080/14779072.2018.1497974.
Cohen HM, Griffiths AD, Tawfik DS, Loakes D. Determinants of cofactor binding to DNA methyltransferases: insights from a systematic series of structural variants of S-adenosylhomocysteine. Org Biomol Chem. 2005 Jan 7;3(1):152-61. doi: 10.1039/b415446k.
Cohen BM, Lipinski JF, Vuckovic A, Prosser E. Blood S-adenosyl-L-methionine levels in psychiatric disorders. Am J Psychiatry. 1982 Feb;139(2):229-31. doi: 10.1176/ajp.139.2.229.
de Vogel S, Dindore V, van Engeland M, Goldbohm RA, van den Brandt PA, Weijenberg MP. Dietary folate, methionine, riboflavin, and vitamin B-6 and risk of sporadic colorectal cancer. J Nutr. 2008 Dec;138(12):2372-8. doi: 10.3945/jn.108.091157.
Gao X, Sanderson SM, Dai Z, Reid MA, Cooper DE, Lu M, Richie JP Jr, Ciccarella A, Calcagnotto A, Mikhael PG, Mentch SJ, Liu J, Ables G, Kirsch DG, Hsu DS, Nichenametla SN, Locasale JW. Dietary methionine influences therapy in mouse cancer models and alters human metabolism. Nature. 2019 Aug;572(7769):397-401. doi: 10.1038/s41586-019-1437-3.
He D, Feng H, Sundberg B, Yang J, Powers J, Christian AH, Wilkinson JE, Monnin C, Avizonis D, Thomas CJ, Friedman RA, Kluger MD, Hollingsworth MA, Grandgenett PM, Klute KA, Toste FD, Chang CJ, Chio IIC. Methionine oxidation activates pyruvate kinase M2 to promote pancreatic cancer metastasis. Mol Cell. 2022 Aug 18;82(16):3045-3060.e11. doi: 10.1016/j.molcel.2022.06.005.
Jung AY, van Duijnhoven FJ, Nagengast FM, Botma A, Heine-Bröring RC, Kleibeuker JH, Vasen HF, Harryvan JL, Winkels RM, Kampman E. Dietary B vitamin and methionine intake and MTHFR C677T genotype on risk of colorectal tumors in Lynch syndrome: the GEOLynch cohort study. Cancer Causes Control. 2014 Sep;25(9):1119-29. doi: 10.1007/s10552-014-0412-4.
Kaiser P. Methionine Dependence of Cancer. Biomolecules. 2020 Apr 8;10(4):568. doi: 10.3390/biom10040568.
Kim HH, Park CS. Methionine cytotoxicity in the human breast cancer cell line MCF-7. In Vitro Cell Dev Biol Anim. 2003 Mar-Apr;39(3-4):117-9. doi: 10.1007/s11626-003-0004-1.
Kune G, Watson L. Colorectal cancer protective effects and the dietary micronutrients folate, methionine, vitamins B6, B12, C, E, selenium, and lycopene. Nutr Cancer. 2006;56(1):11-21. doi: 10.1207/s15327914nc5601_3.
Larsson SC, Giovannucci E, Wolk A. Methionine and vitamin B6 intake and risk of pancreatic cancer: a prospective study of Swedish women and men. Gastroenterology. 2007 Jan;132(1):113-8. doi: 10.1053/j.gastro.2006.10.017.
Levine RL, Mosoni L, Berlett BS, Stadtman ER. Methionine residues as endogenous antioxidants in proteins. Proc Natl Acad Sci U S A. 1996 Dec 24;93(26):15036-40. doi: 10.1073/pnas.93.26.15036.
Li TW, Yang H, Peng H, Xia M, Mato JM, Lu SC. Effects of S-adenosylmethionine and methylthioadenosine on inflammation-induced colon cancer in mice. Carcinogenesis. 2012 Feb;33(2):427-35. doi: 10.1093/carcin/bgr295.
Longnecker DS. Abnormal methyl metabolism in pancreatic toxicity and diabetes. J Nutr. 2002 Aug;132(8 Suppl):2373S-2376S. doi: 10.1093/jn/132.8.2373S. PMID: 12163695.
Lopez MJ, Mohiuddin SS. Biochemistry, Essential Amino Acids. [Updated 2022 Mar 18]. In: StatPearls [Internet]. Treasure Island (FL): StatPearls Publishing; 2022 Jan.
Martinov MV, Vitvitsky VM, Banerjee R, Ataullakhanov FI. The logic of the hepatic methionine metabolic cycle. Biochim Biophys Acta. 2010 Jan;1804(1):89-96. doi: 10.1016/j.bbapap.2009.10.004.
Navik U, Sheth VG, Khurana A, Jawalekar SS, Allawadhi P, Gaddam RR, Bhatti JS, Tikoo K. Methionine as a double-edged sword in health and disease: Current perspective and future challenges. Ageing Res Rev. 2021 Dec;72:101500. doi: 10.1016/j.arr.2021.101500.
Pan Y, Bender PK, Akers RM, Webb KE Jr. Methionine-containing peptides can be used as methionine sources for protein accretion in cultured C2C12 and MAC-T cells. J Nutr. 1996 Jan;126(1):232-41. doi: 10.1093/jn/126.1.232.
Phillips SM. A brief review of critical processes in exercise-induced muscular hypertrophy. Sports Med. 2014 May;44 Suppl 1(Suppl 1):S71-7. doi: 10.1007/s40279-014-0152-3.
Pub Chem [Internet]. Bethesda (MD): National Library of Medicine (US), National Center for Biotechnology Information; 2004-. PubChem Compound Summary for CID 6137, Methionine; [cited 2023 May 26]. Available from: https://pubchem.ncbi.nlm.nih.gov/compound/Methionine
Sedillo JC, Cryns VL. Targeting the methionine addiction of cancer. Am J Cancer Res. 2022 May 15;12(5):2249-2276.
Son P, Lewis L. Hyperhomocysteinemia. [Updated 2022 May 8]. In: StatPearls [Internet]. Treasure Island (FL): StatPearls Publishing; 2023 Jan-. Available from: https://www.ncbi.nlm.nih.gov/books/NBK554408/
Storch KJ, Wagner DA, Burke JF, Young VR. Quantitative study in vivo of methionine cycle in humans using [methyl-2H3]- and [1-13C]methionine. Am J Physiol. 1988 Sep;255(3 Pt 1):E322-31. doi: 10.1152/ajpendo.1988.255.3.E322.
Stoyanov E, Mizrahi L, Olam D, Schnitzer-Perlman T, Galun E, Goldenberg DS. Tumor-suppressive effect of S-adenosylmethionine supplementation in a murine model of inflammation-mediated hepatocarcinogenesis is dependent on treatment longevity. Oncotarget. 2017 May 30;8(62):104772-104784. doi: 10.18632/oncotarget.18300.
Tang Y, Chen X, Chen Q, Xiao J, Mi J, Liu Q, You Y, Chen Y, Ling W. Association of serum methionine metabolites with non-alcoholic fatty liver disease: a cross-sectional study. Nutr Metab (Lond). 2022 Mar 18;19(1):21. doi: 10.1186/s12986-022-00647-7.
Townsend DM, Tew KD, Tapiero H. Sulfur containing amino acids and human disease. Biomed Pharmacother. 2004 Jan;58(1):47-55. doi: 10.1016/j.biopha.2003.11.005.
Tripodi F, Badone B, Vescovi M, Milanesi R, Nonnis S, Maffioli E, Bonanomi M, Gaglio D, Tedeschi G, Coccetti P. Methionine Supplementation Affects Metabolism and Reduces Tumor Aggressiveness in Liver Cancer Cells. Cells. 2020 Nov 16;9(11):2491. doi: 10.3390/cells9112491.
Troen AM, Lutgens E, Smith DE, Rosenberg IH, Selhub J. The atherogenic effect of excess methionine intake. Proc Natl Acad Sci U S A. 2003 Dec 9;100(25):15089-94. doi: 10.1073/pnas.2436385100.
Vidal AC, Grant DJ, Williams CD, Masko E, Allott EH, Shuler K, McPhail M, Gaines A, Calloway E, Gerber L, Chi JT, Freedland SJ, Hoyo C. Associations between Intake of Folate, Methionine, and Vitamins B-12, B-6 and Prostate Cancer Risk in American Veterans. J Cancer Epidemiol. 2012;2012:957467. doi: 10.1155/2012/957467.
Wanders D, Hobson K, Ji X. Methionine Restriction and Cancer Biology. Nutrients. 2020 Mar 3;12(3):684. doi: 10.3390/nu12030684.
Wang L, Alachkar A, Sanathara N, Belluzzi JD, Wang Z, Civelli O. A Methionine-Induced Animal Model of Schizophrenia: Face and Predictive Validity. Int J Neuropsychopharmacol. 2015 May 19;18(12):pyv054. doi: 10.1093/ijnp/pyv054.
Wang Y, Sun Z, Szyf M. S-adenosyl-methionine (SAM) alters the transcriptome and methylome and specifically blocks growth and invasiveness of liver cancer cells. Oncotarget. 2017 Dec 5;8(67):111866-111881. doi: 10.18632/oncotarget.22942.
Waterland RA. Assessing the effects of high methionine intake on DNA methylation. J Nutr. 2006 Jun;136(6 Suppl):1706S-1710S. doi: 10.1093/jn/136.6.1706S.
Wu G, Shi Y, Han L, Feng C, Ge Y, Yu Y, Tang X, Cheng X, Sun J, Le GW. Dietary Methionine Restriction Ameliorated Fat Accumulation, Systemic Inflammation, and Increased Energy Metabolism by Altering Gut Microbiota in Middle-Aged Mice Administered Different Fat Diets. J Agric Food Chem. 2020 Jul 22;68(29):7745-7756. doi: 10.1021/acs.jafc.0c02965.
Wu W, Kang S, Zhang D. Association of vitamin B6, vitamin B12 and methionine with risk of breast cancer: a dose-response meta-analysis. Br J Cancer. 2013 Oct 1;109(7):1926-44. doi: 10.1038/bjc.2013.438.
Xu Y, Yang Y, Li B, Xie Y, Shi Y, Le G. Dietary methionine restriction improves gut microbiota composition and prevents cognitive impairment in D-galactose-induced aging mice. Food Funct. 2022 Dec 13;13(24):12896-12914. doi: 10.1039/d2fo03366f.
Yang D, Baumgartner RN, Slattery ML, Wang C, Giuliano AR, Murtaugh MA, Risendal BC, Byers T, Baumgartner KB. Dietary intake of folate, B-vitamins and methionine and breast cancer risk among Hispanic and non-Hispanic white women. PLoS One. 2013;8(2):e54495. doi: 10.1371/journal.pone.0054495.
Yang Y, Lu M, Xu Y, Qian J, Le G, Xie Y. Dietary Methionine via Dose-Dependent Inhibition of Short-Chain Fatty Acid Production Capacity Contributed to a Potential Risk of Cognitive Dysfunction in Mice. J Agric Food Chem. 2022 Dec 7;70(48):15225-15243. doi: 10.1021/acs.jafc.2c04847.
Yang Y, Lu M, Xu Y, Qian J, Le G, Xie Y. High dietary methionine intake may contribute to the risk of nonalcoholic fatty liver disease by inhibiting hepatic H2S production. Food Res Int. 2022 Aug;158:111507. doi: 10.1016/j.foodres.2022.111507.
Yang Y, Zhang Y, Xu Y, Luo T, Ge Y, Jiang Y, Shi Y, Sun J, Le G. Dietary methionine restriction improves the gut microbiota and reduces intestinal permeability and inflammation in high-fat-fed mice. Food Funct. 2019 Sep 1;10(9):5952-5968. doi: 10.1039/c9fo00766k.
Zhang N. Role of methionine on epigenetic modification of DNA methylation and gene expression in animals. Anim Nutr. 2018 Mar;4(1):11-16. doi: 10.1016/j.aninu.2017.08.009.
Zhou X, He L, Wan D, Yang H, Yao K, Wu G, Wu X, Yin Y. Methionine restriction on lipid metabolism and its possible mechanisms. Amino Acids. 2016 Jul;48(7):1533-40. doi: 10.1007/s00726-016-2247-7.
Zhou ZY, Wan XY, Cao JW. Dietary methionine intake and risk of incident colorectal cancer: a meta-analysis of 8 prospective studies involving 431,029 participants. PLoS One. 2013 Dec 10;8(12):e83588. doi: 10.1371/journal.pone.0083588.