Key Takeaways (expand)
- Short-chain fatty acids (SCFAs) are literally “short”—containing fewer than six carbon atoms in their molecular structure.
- The most common SCFAs are butyric acid, propionic acid, and acetic acid, but others include formic acid, isobutyric acid, valeric acid, isovaleric acid, and 2-methylbutyric acid.
- The majority of SCFAs we absorb are made from the bacterial fermentation of fiber and other carbohydrates by our intestinal microbiota, rather than coming directly from food.
- SCFA-producing gut bacteria mainly belong to the Firmicutes phylum, and they can generate enough SCFAs to contribute about 5 to 15% of our daily calorie intake!
- Short-chain fats have a unique digestive process—getting absorbed directly by colon cells to be used for energy, or passing straight to the liver to become fuel for liver cells.
- Individual SCFAs are preferred by different body tissues: butyric acid is the preferred fuel source for colon cells; propionic acid is most likely to be oxidized in the liver; and acetic acid is the main SCFA to circulate systemically and reach other tissues in the body.
- SCFAs play a central role in gut health—helping maintain gut barrier integrity, improving colonic blood flow, regulating gut motility and gastric emptying, increasing the secretion of antimicrobial peptides, and stimulating the absorption of water and electrolytes by the gut mucosa.
- SCFAs also help our bodies absorb certain minerals, particularly calcium, magnesium, copper, zinc, and iron.
- SCFAs impact the gut-brain axis by helping help cells respond to hormones and neurotransmitters, regulating the passage of nutrients and molecules into the brain, and helping maintain the integrity of the blood-brain barrier itself.
- SCFAs subsequently have wide-ranging effects on neurological health—influencing mood, behavior, and brain physiology.
- SCFAs can even alter the function of satiety hormone in ways that reduce appetite and food intake.
- The actual impact of SCFAs on body weight is less clear, with some studies showing that SCFAs can increase energy expenditure and support weight loss, and others drawing links between higher SCFA levels and obesity.
- The contradictions in SCFA and obesity research may be due to differences in lean versus obese gut microbiota compositions, excess production of SCFAs contributing to total energy availability (AKA extra calories), specific SCFAs having more obesogenic properties than others, or genetic factors.
- Research suggests SCFAs (especially butyric acid) can help protect against colorectal cancer by improving intestinal barrier function, regulating multiple phases of the cell cycle, and reducing tumor cell invasiveness.
- SCFAs may also have a protective role against diabetes, due to modulating hormones involved in insulin secretion, helping regulate blood sugar levels, reducing oxidative stress, and improving beta-cell function in the pancreas.
- Although no SCFA deficiency diseases exist, gut microbiomes that lack sufficient SCFA-producing bacteria are linked with a greater risk of heart failure, high blood pressure, colorectal cancer, diabetes, gout, gut disorders like ulcerative colitis, and altered function of the GI tract.
- Along with the SCFAs produced by our gut bacteria, dietary sources include butter and other high-fat dairy products, lacto-fermented foods (pickles, sauerkraut, soy sauce, yogurt, and some cheeses), vinegar, and alcohol.
- Although still beneficial, diet-derived SCFAs lack some of the features of gut-derived SCFAs, since the process of fermenting fiber into SCFAs reduces gut pH levels and increases the growth and diversity of gut microbiota.
Table of Contents[Hide][Show]
As their name suggests, short-chain fatty acids (or SCFAs) are literally “short,” containing fewer than six carbon atoms in their molecular structure! The most common of these fats are butyric acid, propionic acid, and acetic acid, but others include formic acid, isobutyric acid, valeric acid, isovaleric acid, and 2-methylbutyric acid. You’ll often see SCFAs referred to by their conjugate base names, such as butyrate, propionate, and acetate; this is because in natural environments (such as within the body), these fatty acids quickly donate their protons, transforming them into their conjugate base form. As a result, SCFAs aren’t widely found as “pure” acids, and much of the research conducted on them is actually studying their esters.
SCFAs serve as the main energy source for cells lining the colon, giving them an important role in gastrointestinal health. They also influence appetite and metabolism, mineral absorption, the function of the immune system, and blood glucose and insulin regulation! Through an impact on the gut-brain axis, SCFAs can also affect mood and neurological health.
Unlike most fats, the majority of SCFAs we absorb are actually made from the bacterial fermentation of fiber and other carbohydrates by our intestinal microbiota. However, SCFAs also naturally occur in some foods. For instance, high-fat dairy products like butter are rich in butyric acid, which is produced by ruminant animals’ own fermentation process (in fact, the word “butyric” comes from the Ancient Greek word for “butter”—the substance it was first discovered in!). Lacto-fermented foods such as pickles, sauerkraut, soy sauce, yogurt, and some cheeses (Emmental, Gruyere, Swiss, Comté, and hard Italian cheeses like Grana, Padano, and Parmigiano Reggiano) contain propionic acid and acetic acid, which are produced when bacteria convert the lactates generated by starter cultures into SCFAs. Additionally, vinegar and alcohol contain acetic acid.
Everything You Need to Jump into Nutrivore TODAY!
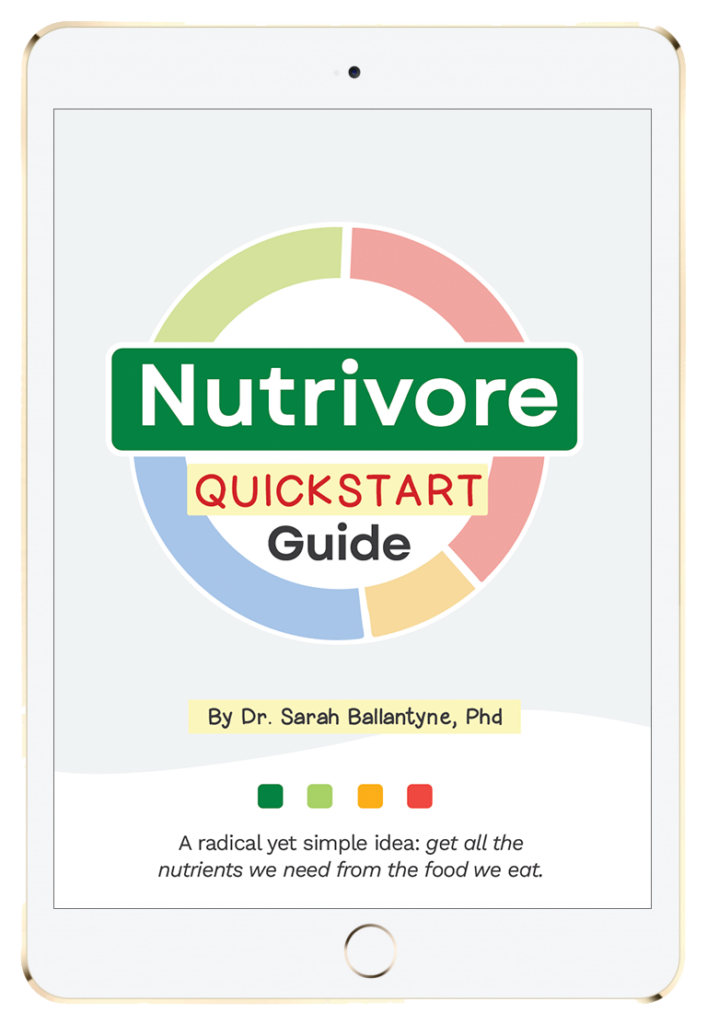
Nutrivore Quickstart Guide
The Nutrivore Quickstart Guide e-book explains why and how to eat a Nutrivore diet, introduces the Nutrivore Score, gives a comprehensive tour of the full range of essential and important nutrients!
Plus, you’ll find the Top 100 Nutrivore Score Foods, analysis of food groups, practical tips to increase the nutrient density of your diet, and look-up tables for the Nutrivore Score of over 700 foods.
Buy now for instant digital access.
The Biological Roles of Short-Chain Fatty Acids
The human body obtains SCFAs in one of two ways: either directly from foods, or through microbial fermentation in the gut. More specifically, certain gut bacteria (mostly from the Firmicutes phylum) ferment carbohydrates that escape digestion and absorption in the small intestine—including resistant starch, inulin, fructo-oligosaccharides, sugar alcohols, and the polysaccharides from plant cell walls, such as cellulose. When these carbohydrates reach the large intestine, carbohydrate-degrading bacteria take part in fermentation pathways that produce SCFAs along with other byproducts like carbon dioxide, hydrogen, and methane. By some estimates, our gut bacteria ferment about 50 to 60 g of carbohydrates each day, producing enough SCFAs to contribute about 5 to 15% of our daily calorie intake (depending on factors like total carbohydrate intake, fiber intake, and the composition of the gut microbiota). The vast majority of the SCFAs in the gut (90 to 95%) are acetic acid, butyric acid, and propionic acid, existing in a 60/20/20 ratio, respectively.
In contrast to long-chain fatty acids, which are transported via large lipoprotein particles called chylomicrons, short-chain fats get absorbed directly by colon cells, where they’re then metabolized and used for energy (in fact, SCFAs provide about 60 to 70% of the energy used by colon epithelial cells, AKA colonocytes). The SCFAs that don’t get used by colon cells get taken up through the portal vein (a blood vessel that carries blood from the GI tract to the liver), where they can become fuel for liver cells. Only a very small amount of SCFAs end up in systemic circulation and reach other tissues.
Within the body, these special fats serve a number of important functions! For one, they play a central role in the health of the gut, and regulate a variety of processes occurring there. Along with helping maintain gut barrier integrity (mostly by modifying tight junction protein and mucin expression), short-chain fatty acids improve colonic blood flow, help regulate gut motility and gastric emptying, stimulate the absorption of water and electrolytes by the gut mucosa, and increase the secretion of antimicrobial peptides by epithelial cells. They also aid in the absorption of minerals such as calcium, magnesium, copper, zinc, and iron. Interestingly, many of the gut health benefits attributed to fiber consumption are actually due to the production of these short-chain fatty acids!
SCFAs can also impact the gut-brain axis—the two-way communication loop between the GI tract and the central nervous system. For example, circulating short-chain fats can cross the blood-brain barrier and interact with certain membrane proteins (called G protein-coupled receptors) that help cells respond to hormones and neurotransmitters. These fats also seem to help maintain the integrity of the blood-brain barrier itself (possibly by altering the expression of tight junction proteins like claudin and occludin), giving them a role in maintaining central nervous system homeostasis and helping regulate the passage of nutrients and molecules from circulation to the brain. As a result, SCFAs can have wide-ranging effects on neurological health—potentially influencing mood, behavior, and brain physiology. SCFAs can even alter hormone function in ways that affect appetite and food intake, by stimulating receptors associated with two appetite-suppressing hormones: glucagon-like peptide-1 (GLP-1), and the peptide YY (PYY), both of which signal feelings of satiety.
Although these fats have a number of similarities on the whole, individual SCFAs possess some distinct qualities. For example, butyrate plays a particularly important role in gut health and cellular energy metabolism. Out of all the short-chain fats, it’s the most preferred fuel source for colonocytes: about 70 to 90% of the butyrate in the GI tract gets taken up as energy for colon cells. Butyrate also seems to have the most prominent role in modulating intestinal barrier function, immune responses (including to invasive pathogens in the gut), and inflammatory responses within the gut, along with promoting epithelial cell growth. Across a variety of studies, it’s been shown to promote the differentiation of regulatory T-cells, giving it a major role in suppressing inflammation and allergic responses.
Meanwhile, compared to butyrate, a relatively higher amount of propionate reaches the liver, where it contributes to gluconeogenesis. Priopionate also appears particularly effective at reducing lipid concentrations in the blood and liver, and has been shown to inhibit cholesterol synthesis. Acetate (which can’t be oxidized in the liver) is the most likely SCFA to make it all the way into systemic circulation.
Butyrate and propionate (less so acetate) are also inhibitors of histone deacetylase—an enzyme that decreases gene expression by altering the histone proteins on DNA. In general, histone deacetylase inhibitors have significant anti-inflammatory activity and anti-cancer properties, including inducing cell cycle arrest and death of malignant cells.
It’s worth noting that SCFAs coming directly from diet and SCFAs produced by gut bacteria can impact the body in different ways. For example, the process of fermenting fiber into SCFAs decreases the pH levels of the gut, increases the growth and diversity of gut microbiota, and increases fecal acidification—all of which directly contribute to gut health (and indirectly contribute to the health of other body systems connected to the gut!). These changes don’t seem to occur when consuming preformed SCFAs. Unfortunately, very little research exists on the effects of dietary SCFAs versus gut-generated SCFAs, so it’s hard to say how dramatically these differences impact human health on the whole.
Want to Know ALL the Easy Steps to Nutrivore?
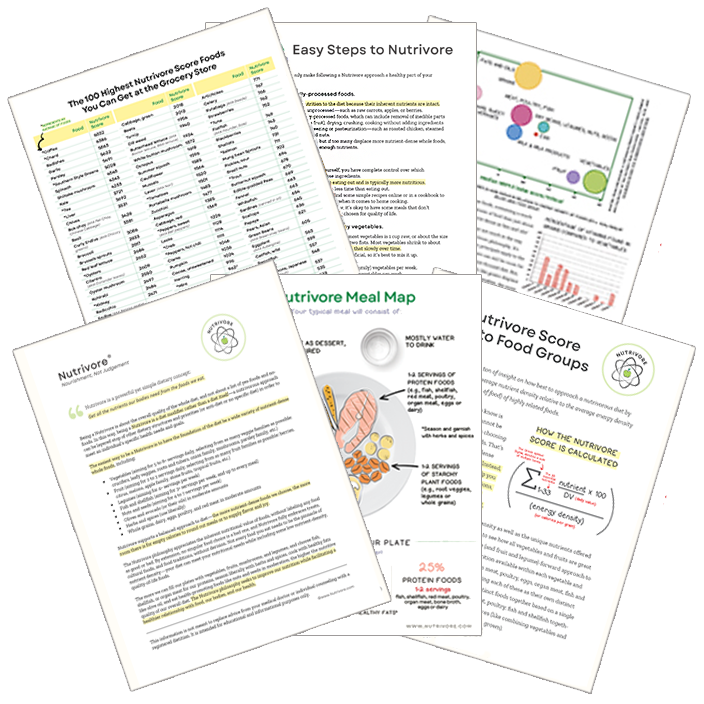
Get it Directly in Your Inbox!
The Nutrivore Newsletter is a weekly email that delivers bite-size fun facts, practical tips, recipes and resources. Sign up now and get 5 free guides directly to your inbox:
- Easy Steps to Nutrivore 4-page guide
- Nutrivore Foundational Foods 6-page guide
- Nutrivore Score Guide to Food Groups 3-page guide
- Nutrivore Meal Map
- Top 100 Nutrivore Score Foods
Short-Chain Fatty Acids in Health and Disease
Evidence suggests that SCFAs could help protect against a number of diseases and health conditions—including cancer, inflammatory gut disorders, diabetes, cardiovascular disease, obesity, and even neurological conditions!
SCFAs and Cancer
SCFAs have been studied for their role in protecting against colorectal cancer, with butyrate showing the strongest inhibitory effect out of all the SCFAs. Largely through its activity as a histone deacetylation inhibitor, butyrate can promote cell differentiation, induce cell cycle arrest, promote the death (apoptosis) of transformed colonocytes, inhibit cell proliferation, reduce tumor cell invasiveness, and decrease the transformation of primary to secondary bile acids. Similarly, colorectal cancer in general is strongly linked to intestinal barrier dysfunction (which activates tumor-associated macrophages and inflammatory molecules), and one of butyrate’s effects is maintaining the integrity of this barrier.
Butyrate also influences the central nervous system in a way that increases gut motility, which contributes additional protection against colorectal cancer. There’s even some evidence that butyrate prevents the growth and enhances the death of other types of cancer cells, such as bladder!
And while less research has been conducted on the other SCFAs in relation to cancer, in vitro studies suggest that propionic acid could inhibit the growth of cervical cancer and lung cancer cells.
SCFAs and Gut Health
Several studies have also been conducted on SCFAs (particularly butyrate) and inflammatory gut diseases. One randomized controlled trial showed that butyrate supplementation (300 mg daily for four weeks) significantly reduced abdominal pain in patients with irritable bowel syndrome. In another study, 4 g of butyrate daily for a total of eight weeks led to a significant improvement in Crohn’s disease symptoms.
The THREE Best Probiotics
Bacillus/Spore-based
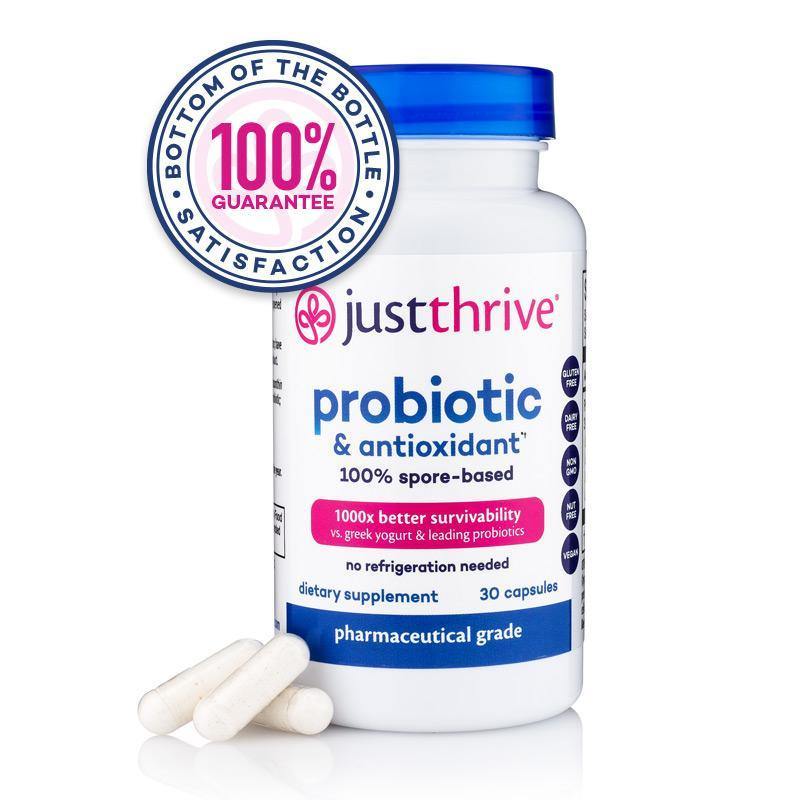
Best for a general probiotic, if you were recently on antibiotics, or for digestion issues (especially abdominal pain, bloating, flatulence, diarrhea, and gas after meals).
Lactobacillus & Bifidobacterium
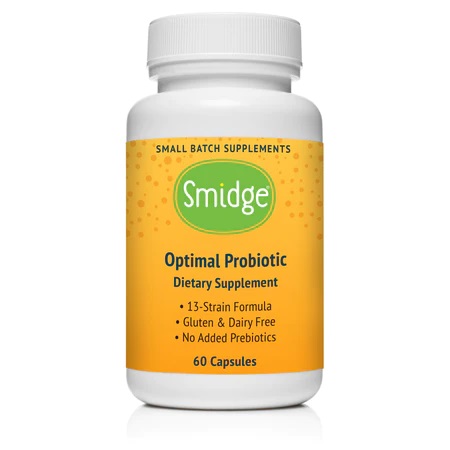
Best for if you don’t eat fermented foods, if you were recently on antibiotics, or if you have issues with constipation or gas.
Saccharomyces boulardii
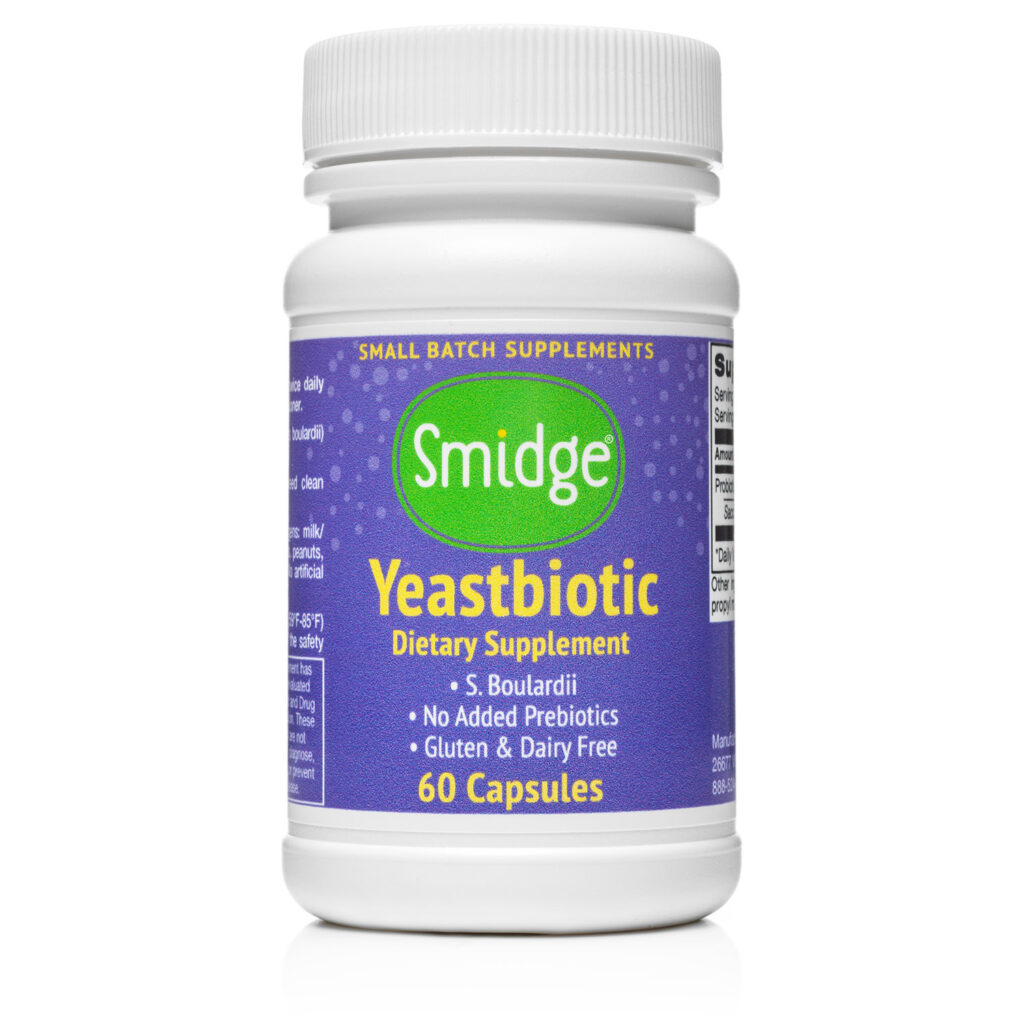
Best for if you have Candida issues, were recently on antibiotics, or have diarrhea.
SCFAs and Type 2 Diabetes
SCFAs may have a role in protecting against diabetes. For one, they help regulate blood sugar concentrations through their effects on hormones that mediate insulin secretion (such as GLP-1). A variety of studies have shown that SCFAs can also decrease glycolysis (the breakdown of glucose) and gluconeogenesis (the synthesis of glucose) in the liver, increase the body’s abundance of glucose transporters (which facilitate the movement of glucose into cells), and reduce oxidative stress (which is known to interrupt insulin signaling and promote insulin resistance).
In rodent models of diet-induced diabetes, butyrate supplementation was able to protect against high blood sugar and insulin resistance, in part by promoting more energy expenditure, stimulating mitochondrial function in skeletal muscle, and preventing weight gain.
In humans, there’s observational evidence linking diabetes with low levels of SCFA-producing bacteria in the gut, and a trial of propionate supplementation (in the form of an inulin-propionate ester) for 24 weeks led to improvements in pancreatic beta-cell function. Other human studies suggest that acetate—particularly coming from vinegar—has potent blood sugar-lowering properties, with an ability to reduce fasting blood glucose, enhance insulin sensitivity, and reduce the blood sugar and insulin response to high-carbohydrate meals.
However, controlled human trials are in short supply on this topic, so more research is needed!
SCFAs and Cardiovascular Disease
SCFAs have also been implicated in various aspects of cardiovascular health. Research shows that SCFAs exhibit many heart-relevant biological activities affecting blood sugar regulation, lipid and glucose metabolism, inflammation, neural signaling, oxidative stress, and epigenetic modulation.
In mouse models of heart disease, for example, butyrate supplementation (at 1% of the total diet) was able to protect against atherosclerotic lesions, largely by decreasing the production of adhesion molecules and reducing macrophage migration; butyrate supplementation (200 to 400 mg per kg) in mice likewise lowered the animals’ cholesterol levels, due to butyrate’s effects on bile acid metabolism and cholesterol elimination. Other studies in rodents and in vitro have shown that butyrate can directly protect the integrity and architecture of cardiac tissue, due to lowering uric acid levels and enhancing antioxidant defenses, while also reducing the cellular uptake of oxidized LDL (a step in atherosclerotic plaque creation). SCFAs can even help combat hypertension, with animal studies showing that butyrate supplementation reduces both systolic and diastolic blood pressure—in part by modulating the expression of free fatty acid receptor 3 (which contributes to vascular tone and blood vessel dilation) and increasing cyclic AMP levels (which is a second messenger in blood pressure control). And although the findings haven’t been totally consistent across studies, some animal research suggests that butyrate supplementation can reduce triglyceride levels and total cholesterol.
More controlled trials in humans will be helpful for further clarifying the relationship between SCFAs and cardiovascular health!
SCFAs and Neurological and Neurodegenerative Diseases
Growing evidence also suggests a role of SCFAs in central nervous system health, potentially influencing brain physiology, behavior, and possibly even neurodegenerative diseases.
Dietary intervention studies in humans suggest that SCFAs can indirectly mediate mood and emotion through their effects on neurotransmitters and neuroinflammation, and mouse models of Alzheimer’s disease have shown that some SCFAs (particularly butyrate) can help preserve memory function and learning capacity. Likewise, animal models of mania show that butyrate supplementation can reverse behavioral hyperactivity. Observational studies in humans also reveal links between SCFAs and neurodegenerative diseases: people with Parkinson’s disease, for instance, have significantly lower levels of SCFA-producing bacteria in their guts (relative to healthy individuals), while children with autism have altered fecal levels of SCFAs.
However, very few human trials are available on this topic, and the ones that do exist don’t have the most robust methodology. So, once again, more research is needed!
SCFAs and Obesity
When it comes to obesity, SCFAs food intake, and body weight have a complex relationship, and scientists are still trying to untangle some contradictions that have emerged from existing research. On one hand, SCFAs have clear mechanisms by which they can suppress appetite and food intake—namely by increasing levels of satiety hormones (GLP-1 and peptide YY) in the body. Propionate and acetate have also been shown increase the secretion of leptin, a hormone that helps regulate body weight. SCFAs also have the potential to increase energy expenditure through their effects on different metabolic pathways. In animal models, for example, butyrate supplementation was able to enhance adaptive thermogenesis and fat oxidation, effectively counteracting the effects of diets designed to induce obesity. And in mice fed a high-fat diet, adding sodium butyrate (as 5% of the total diet by weight) allowed the animals to maintain their body fat levels within a healthy range without any reduction in food intake. Additional rodent studies show that butyrate suppresses food intake and protects against diet-induced obesity, elevated insulin, high triglycerides, and fatty liver, while also boosting fat oxidation by activating brown adipose tissue (a special type of body fat that enhances energy expenditure by producing heat). And while controlled human trials are harder to come by, some interventions suggest that acetate supplementation (in the form of vinegar) could significantly reduce body weight in obese humans, along with lowering blood sugar levels.
However, other research seems to contradict an anti-obesity effect of SCFAs! Some studies of lean versus obese individuals show that obesity is associated with higher levels of SCFAs (typically measured in the feces), and that SCFA levels go down after the obesity is treated (AKA weight loss). These findings appear due to differences in lean versus obese gut microbiota compositions, with the latter producing more SCFAs on the whole. Likewise, observational studies measuring fasting acetate levels have been highly inconsistent, with some showing a negative association with body fat mass, some showing a positive association, and some showing that higher acetate levels are predictive of greater weight gain over time. And in both animal models and controlled human trials, dietary propionate has been shown to promote high blood sugar and weight gain (mostly through endocrine effects), as well as promote elevated blood sugar, insulin resistance, and hyperinsulinemia. The metabolic-disruptive effects of propionate were actually demonstrated as early as the year 1912, when a study found that administering propionate to dogs increased their glucose production! These are important findings, because propionate is often used as a food preservative due to its ability to inhibit mold, and the levels shown to promote insulin resistance in humans are equivalent to real-world intakes.
There could be a number of reasons behind these inconsistent findings. For one, SCFA levels in the feces might not accurately reflect total SCFA availability, since such a large portion of SCFAs (up to 95%) get absorbed before excretion; this makes observational correlations between fecal SCFAs and obesity potentially unreliable. It’s also possible that while certain ranges of SCFAs have a net benefit for body weight regulation (through their hormonal and metabolic effects), excess SCFAs simply increase total energy availability, leading to more fat storage. Researchers have even found that certain genetic variations influence the levels of some SCFAs in the gut (particularly butyrate and propionate), and it’s possible that a host gene/gut microbiota interaction could cause an overproduction of SCFAs that leads to calorie surplus. SCFAs that aren’t used by colonocytes can also become substrates for glucose and lipid production: in a stable isotope experiment where SCFAs were infused directly into the colons of mice, up to 62% of infused propionate ended up being used for whole-body glucose production, while infused acetate and butyrate contributed to cholesterol and saturated fat synthesis in the liver. A stable isotope study of SCFAs infusions into the colons of healthy human adults similarly found that smaller, but still significant, amounts of acetate and propionate were used for fatty acid and glucose production, respectively.
Additionally, because individual SCFAs vary in their health effects, the relative abundance of different SCFAs could help explain these research paradoxes. Studies looking at individual SCFAs (rather than just total SCFAs) have shown that obese individuals have a higher proportion of propionate, specifically, than lean individuals, suggesting that propionate could be uniquely obesogenic among the SCFAs.
There’s even evidence that SCFAs have different effects depending on where they’re present in the GI tract! For example, in obese humans, infusions of SCFAs into the distal colon (closest to the rectum) increased fat oxidation and energy expenditure, but SCFA infusions into the proximal colon (the first and middle parts of the colon) failed to produce these same benefits. This could mean that the location of SCFA-producing bacteria in the gut (and therefore, where SCFAs become most highly concentrated) is a relevant issue when it comes to how they affect body weight. Distribution of SCFA-producing bacteria could be influenced by genetics, various lifestyle factors, diet, and/or the environment of the colon—such as pH levels or mineral availability, which vary throughout the span of the GI tract and impact which bacteria can take up residence.
Unfortunately, a shortage of controlled human studies on SCFAs and obesity makes it very hard to get to the bottom of these inconsistencies. For now, most evidence suggests that diets likely to generate abundant SCFAs (e.g., high in resistant starches and fiber) have beneficial effects for weight maintenance and health in general. Clearly, much more research is needed to explore the relationship between SCFAs and body weight in humans!
Didn’t know SCFAs were this amazing? Maybe your friends will enjoy this too!
Health Effects of Short-Chain Fatty Acids Deficiency
Because short-chain fatty acids aren’t biologically essential, there are no true deficiency diseases associated with them. However, low levels of short-chain fats in the gut—as well as low levels of the bacteria that produce them—have been associated with a number of gut issues and adverse health outcomes.
For example, gut microbiomes that lack sufficient short chain fatty acid-producing microorganisms have been linked with a greater risk of heart failure, high blood pressure, colorectal cancer, diabetes, gout, and gut disorders like ulcerative colitis. Low levels of these bacteria and the SCFAs they generate can also alter the function of the GI tract, leading to reduced gut motility (AKA longer transit times) and irregularity.
How Much Short-Chain Fatty Acids Do We Need?
Due to their non-essential nature, and the fact that our bodies produce the majority of the SCFAs available to us, there is no dietary recommendation for daily SCFA intake. However, consuming a varied, high-fiber diet containing resistant starch, as well as lacto-fermented foods, is a great way to increase the availability of SCFAs to our bodies. Likewise, maintaining a diet and lifestyle supportive of gut health helps preserve SCFA-producing bacteria in our microbiomes.
Easily track your servings of Nutrivore Foundational Foods!
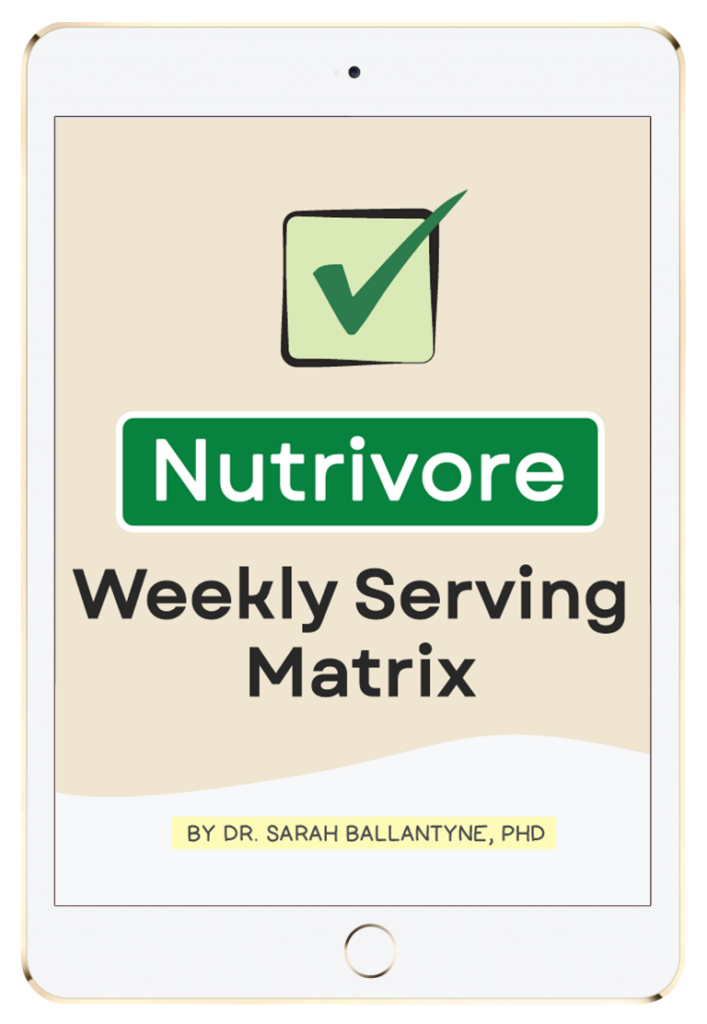
The Nutrivore Weekly Serving Matrix
The Nutrivore Weekly Serving Matrix digital resource is an easy-to-use and flexible weekly checklist designed to help you maximize nutrient-density and meet serving suggestions of Nutrivore foundational foods, all without having to weigh or measure your foods!
Includes a 22-page instructional guide and downloadable interactive guides.
Buy now for instant digital access.
Citations
Expand to see all scientific references for this article.
Adler GK, Hornik ES, Murray G, Bhandari S, Yadav Y, Heydarpour M, Basu R, Garg R, Tirosh A. Acute effects of the food preservative propionic acid on glucose metabolism in humans. BMJ Open Diabetes Res Care. 2021 Jul;9(1):e002336. doi: 10.1136/bmjdrc-2021-002336.
Aguilar EC, da Silva JF, Navia-Pelaez JM, Leonel AJ, Lopes LG, Menezes-Garcia Z, Ferreira AVM, Capettini LDSA, Teixeira LG, Lemos VS, Alvarez-Leite JI. Sodium butyrate modulates adipocyte expansion, adipogenesis, and insulin receptor signaling by upregulation of PPAR-γ in obese Apo E knockout mice. Nutrition. 2018 Mar;47:75-82. doi: 10.1016/j.nut.2017.10.007.
Amiri P, Hosseini SA, Ghaffari S, Tutunchi H, Ghaffari S, Mosharkesh E, Asghari S, Roshanravan N. Role of Butyrate, a Gut Microbiota Derived Metabolite, in Cardiovascular Diseases: A comprehensive narrative review. Front Pharmacol. 2022 Feb 2;12:837509. doi: 10.3389/fphar.2021.837509.
Banasiewicz T, Krokowicz Ł, Stojcev Z, Kaczmarek BF, Kaczmarek E, Maik J, Marciniak R, Krokowicz P, Walkowiak J, Drews M. Microencapsulated sodium butyrate reduces the frequency of abdominal pain in patients with irritable bowel syndrome. Colorectal Dis. 2013 Feb;15(2):204-9. doi: 10.1111/j.1463-1318.2012.03152.x.
Boets E, Deroover L, Houben E, Vermeulen K, Gomand SV, Delcour JA, Verbeke K. Quantification of in Vivo Colonic Short Chain Fatty Acid Production from Inulin. Nutrients. 2015 Oct 28;7(11):8916-29. doi: 10.3390/nu7115440.
Canfora EE, Jocken JW, Blaak EE. Short-chain fatty acids in control of body weight and insulin sensitivity. Nat Rev Endocrinol. 2015 Oct;11(10):577-91. doi: 10.1038/nrendo.2015.128.
Chen G, Ran X, Li B, Li Y, He D, Huang B, Fu S, Liu J, Wang W. Sodium Butyrate Inhibits Inflammation and Maintains Epithelium Barrier Integrity in a TNBS-induced Inflammatory Bowel Disease Mice Model. EBioMedicine. 2018 Apr;30:317-325. doi: 10.1016/j.ebiom.2018.03.030.
Cheng Y, Liu J, Ling Z. Short-chain fatty acids-producing probiotics: A novel source of psychobiotics. Crit Rev Food Sci Nutr. 2022;62(28):7929-7959. doi: 10.1080/10408398.2021.1920884.
den Besten G, Lange K, Havinga R, van Dijk TH, Gerding A, van Eunen K, Müller M, Groen AK, Hooiveld GJ, Bakker BM, Reijngoud DJ. Gut-derived short-chain fatty acids are vividly assimilated into host carbohydrates and lipids. Am J Physiol Gastrointest Liver Physiol. 2013 Dec;305(12):G900-10. doi: 10.1152/ajpgi.00265.2013.
den Besten G, van Eunen K, Groen AK, Venema K, Reijngoud DJ, Bakker BM. The role of short-chain fatty acids in the interplay between diet, gut microbiota, and host energy metabolism. J Lipid Res. 2013 Sep;54(9):2325-40. doi: 10.1194/jlr.R036012. Epub 2013 Jul 2. PMID: 23821742; PMCID: PMC3735932.
Di Sabatino A, Morera R, Ciccocioppo R, Cazzola P, Gotti S, Tinozzi FP, Tinozzi S, Corazza GR. Oral butyrate for mildly to moderately active Crohn’s disease. Aliment Pharmacol Ther. 2005 Nov 1;22(9):789-94. doi: 10.1111/j.1365-2036.2005.02639.x.
Fukumoto S, Tatewaki M, Yamada T, Fujimiya M, Mantyh C, Voss M, Eubanks S, Harris M, Pappas TN, Takahashi T. Short-chain fatty acids stimulate colonic transit via intraluminal 5-HT release in rats. Am J Physiol Regul Integr Comp Physiol. 2003 May;284(5):R1269-76. doi: 10.1152/ajpregu.00442.2002.
Gao Z, Yin J, Zhang J, Ward RE, Martin RJ, Lefevre M, Cefalu WT, Ye J. Butyrate improves insulin sensitivity and increases energy expenditure in mice. Diabetes. 2009 Jul;58(7):1509-17. doi: 10.2337/db08-1637.
Hernández MAG, Canfora EE, Jocken JWE, Blaak EE. The Short-Chain Fatty Acid Acetate in Body Weight Control and Insulin Sensitivity. Nutrients. 2019 Aug 18;11(8):1943. doi: 10.3390/nu11081943.
Holmes ZC, Silverman JD, Dressman HK, Wei Z, Dallow EP, Armstrong SC, Seed PC, Rawls JF, David LA. Short-Chain Fatty Acid Production by Gut Microbiota from Children with Obesity Differs According to Prebiotic Choice and Bacterial Community Composition. mBio. 2020 Aug 11;11(4):e00914-20. doi: 10.1128/mBio.00914-20.
Hong J, Jia Y, Pan S, Jia L, Li H, Han Z, Cai D, Zhao R. Butyrate alleviates high fat diet-induced obesity through activation of adiponectin-mediated pathway and stimulation of mitochondrial function in the skeletal muscle of mice. Oncotarget. 2016 Aug 30;7(35):56071-56082. doi: 10.18632/oncotarget.11267.
Hosseini E, Grootaert C, Verstraete W, Van de Wiele T. Propionate as a health-promoting microbial metabolite in the human gut. Nutr Rev. 2011 May;69(5):245-58. doi: 10.1111/j.1753-4887.2011.00388.x.
Johnston CS, Buller AJ. Vinegar and peanut products as complementary foods to reduce postprandial glycemia. J Am Diet Assoc. 2005 Dec;105(12):1939-42. doi: 10.1016/j.jada.2005.07.012.
Kim K, Kwon O, Ryu TY, Jung CR, Kim J, Min JK, Kim DS, Son MY, Cho HS. Propionate of a microbiota metabolite induces cell apoptosis and cell cycle arrest in lung cancer. Mol Med Rep. 2019 Aug;20(2):1569-1574. doi: 10.3892/mmr.2019.10431.
Kobayashi M, Mikami D, Kimura H, Kamiyama K, Morikawa Y, Yokoi S, Kasuno K, Takahashi N, Taniguchi T, Iwano M. Short-chain fatty acids, GPR41 and GPR43 ligands, inhibit TNF-α-induced MCP-1 expression by modulating p38 and JNK signaling pathways in human renal cortical epithelial cells. Biochem Biophys Res Commun. 2017 Apr 29;486(2):499-505. doi: 10.1016/j.bbrc.2017.03.071.
Kondo T, Kishi M, Fushimi T, Ugajin S, Kaga T. Vinegar intake reduces body weight, body fat mass, and serum triglyceride levels in obese Japanese subjects. Biosci Biotechnol Biochem. 2009 Aug;73(8):1837-43. doi: 10.1271/bbb.90231.
Li Z, Yi CX, Katiraei S, Kooijman S, Zhou E, Chung CK, Gao Y, van den Heuvel JK, Meijer OC, Berbée JFP, Heijink M, Giera M, Willems van Dijk K, Groen AK, Rensen PCN, Wang Y. Butyrate reduces appetite and activates brown adipose tissue via the gut-brain neural circuit. Gut. 2018 Jul;67(7):1269-1279. doi: 10.1136/gutjnl-2017-314050.
Liu H, Wang J, He T, Becker S, Zhang G, Li D, Ma X. Butyrate: A Double-Edged Sword for Health? Adv Nutr. 2018 Jan 1;9(1):21-29. doi: 10.1093/advances/nmx009.
Louis P, Flint HJ. Formation of propionate and butyrate by the human colonic microbiota. Environ Microbiol. 2017 Jan;19(1):29-41. doi: 10.1111/1462-2920.13589. Epub 2016 Dec 8. PMID: 27928878.
Luu M, Weigand K, Wedi F, Breidenbend C, Leister H, Pautz S, Adhikary T, Visekruna A. Regulation of the effector function of CD8+ T cells by gut microbiota-derived metabolite butyrate. Sci Rep. 2018 Sep 26;8(1):14430. doi: 10.1038/s41598-018-32860-x.
Martin-Gallausiaux C, Marinelli L, Blottière HM, Larraufie P, Lapaque N. SCFA: mechanisms and functional importance in the gut. Proc Nutr Soc. 2021 Feb;80(1):37-49. doi: 10.1017/S0029665120006916.
McNabney SM, Henagan TM. Short Chain Fatty Acids in the Colon and Peripheral Tissues: A Focus on Butyrate, Colon Cancer, Obesity and Insulin Resistance. Nutrients. 2017 Dec 12;9(12):1348. doi: 10.3390/nu9121348.
Montel MC, Buchin S, Mallet A, Delbes-Paus C, Vuitton DA, Desmasures N, Berthier F. Traditional cheeses: rich and diverse microbiota with associated benefits. Int J Food Microbiol. 2014 May 2;177:136-54. doi: 10.1016/j.ijfoodmicro.2014.02.019.
Morris A. Metabolic safety of common preservative under scrutiny. Nat Rev Endocrinol. 2019 Jul;15(7):378. doi: 10.1038/s41574-019-0217-3.
Nogal A, Valdes AM, Menni C. The role of short-chain fatty acids in the interplay between gut microbiota and diet in cardio-metabolic health. Gut Microbes. 2021 Jan-Dec;13(1):1-24. doi: 10.1080/19490976.2021.1897212.
Pham CH, Lee JE, Yu J, Lee SH, Yu KR, Hong J, Cho N, Kim S, Kang D, Lee S, Yoo HM. Anticancer Effects of Propionic Acid Inducing Cell Death in Cervical Cancer Cells. Molecules. 2021 Aug 16;26(16):4951. doi: 10.3390/molecules26164951.
Pingitore A, Chambers ES, Hill T, Maldonado IR, Liu B, Bewick G, Morrison DJ, Preston T, Wallis GA, Tedford C, Castañera González R, Huang GC, Choudhary P, Frost G, Persaud SJ. The diet-derived short chain fatty acid propionate improves beta-cell function in humans and stimulates insulin secretion from human islets in vitro. Diabetes Obes Metab. 2017 Feb;19(2):257-265. doi: 10.1111/dom.12811.
Portincasa P, Bonfrate L, Vacca M, De Angelis M, Farella I, Lanza E, Khalil M, Wang DQ, Sperandio M, Di Ciaula A. Gut Microbiota and Short Chain Fatty Acids: Implications in Glucose Homeostasis. Int J Mol Sci. 2022 Jan 20;23(3):1105. doi: 10.3390/ijms23031105.
Rahat-Rozenbloom S, Fernandes J, Gloor GB, Wolever TM. Evidence for greater production of colonic short-chain fatty acids in overweight than lean humans. Int J Obes (Lond). 2014 Dec;38(12):1525-31. doi: 10.1038/ijo.2014.46.
Ringer A. The quantitative conversion of propionic acid into glucose. J Biol Chem. 1912;12(3):511-5.
Ríos-Covián D, Ruas-Madiedo P, Margolles A, Gueimonde M, de Los Reyes-Gavilán CG, Salazar N. Intestinal Short Chain Fatty Acids and their Link with Diet and Human Health. Front Microbiol. 2016 Feb 17;7:185. doi: 10.3389/fmicb.2016.00185.
Schönfeld P, Wojtczak L. Short- and medium-chain fatty acids in energy metabolism: the cellular perspective. J Lipid Res. 2016 Jun;57(6):943-54. doi: 10.1194/jlr.R067629.
Schwiertz A, Taras D, Schäfer K, Beijer S, Bos NA, Donus C, Hardt PD. Microbiota and SCFA in lean and overweight healthy subjects. Obesity (Silver Spring). 2010 Jan;18(1):190-5. doi: 10.1038/oby.2009.167.
Shimizu H, Masujima Y, Ushiroda C, Mizushima R, Taira S, Ohue-Kitano R, Kimura I. Dietary short-chain fatty acid intake improves the hepatic metabolic condition via FFAR3. Sci Rep. 2019 Nov 12;9(1):16574. doi: 10.1038/s41598-019-53242-x.
Sieuwerts S, de Bok FA, Hugenholtz J, van Hylckama Vlieg JE. Unraveling microbial interactions in food fermentations: from classical to genomics approaches. Appl Environ Microbiol. 2008 Aug;74(16):4997-5007. doi: 10.1128/AEM.00113-08.
Silva YP, Bernardi A, Frozza RL. The Role of Short-Chain Fatty Acids From Gut Microbiota in Gut-Brain Communication. Front Endocrinol (Lausanne). 2020 Jan 31;11:25. doi: 10.3389/fendo.2020.00025.
Tirosh A, Calay ES, Tuncman G, Claiborn KC, Inouye KE, Eguchi K, Alcala M, Rathaus M, Hollander KS, Ron I, Livne R, Heianza Y, Qi L, Shai I, Garg R, Hotamisligil GS. The short-chain fatty acid propionate increases glucagon and FABP4 production, impairing insulin action in mice and humans. Sci Transl Med. 2019 Apr 24;11(489):eaav0120. doi: 10.1126/scitranslmed.aav0120.
Turnbaugh PJ, Ley RE, Mahowald MA, Magrini V, Mardis ER, Gordon JI. An obesity-associated gut microbiome with increased capacity for energy harvest. Nature. 2006 Dec 21;444(7122):1027-31. doi: 10.1038/nature05414.
van der Beek CM, Canfora EE, Lenaerts K, Troost FJ, Damink SWMO, Holst JJ, Masclee AAM, Dejong CHC, Blaak EE. Distal, not proximal, colonic acetate infusions promote fat oxidation and improve metabolic markers in overweight/obese men. Clin Sci (Lond). 2016 Nov 1;130(22):2073-2082. doi: 10.1042/CS20160263.
van der Beek CM, Dejong CHC, Troost FJ, Masclee AAM, Lenaerts K. Role of short-chain fatty acids in colonic inflammation, carcinogenesis, and mucosal protection and healing. Nutr Rev. 2017 Apr 1;75(4):286-305. doi: 10.1093/nutrit/nuw067.
Waldecker M, Kautenburger T, Daumann H, Busch C, Schrenk D. Inhibition of histone-deacetylase activity by short-chain fatty acids and some polyphenol metabolites formed in the colon. J Nutr Biochem. 2008 Sep;19(9):587-93. doi: 10.1016/j.jnutbio.2007.08.002.
Walker AW, Duncan SH, McWilliam Leitch EC, Child MW, Flint HJ. pH and peptide supply can radically alter bacterial populations and short-chain fatty acid ratios within microbial communities from the human colon. Appl Environ Microbiol. 2005 Jul;71(7):3692-700. doi: 10.1128/AEM.71.7.3692-3700.2005.
Wong JM, de Souza R, Kendall CW, Emam A, Jenkins DJ. Colonic health: fermentation and short chain fatty acids. J Clin Gastroenterol. 2006 Mar;40(3):235-43. doi: 10.1097/00004836-200603000-00015.
Wu X, Wu Y, He L, Wu L, Wang X, Liu Z. Effects of the intestinal microbial metabolite butyrate on the development of colorectal cancer. J Cancer. 2018 Jun 15;9(14):2510-2517. doi: 10.7150/jca.25324.
Yang Q, Ouyang J, Sun F, Yang J. Short-Chain Fatty Acids: A Soldier Fighting Against Inflammation and Protecting From Tumorigenesis in People With Diabetes. Front Immunol. 2020 Dec 8;11:590685. doi: 10.3389/fimmu.2020.590685.
Zeng H, Hamlin SK, Safratowich BD, Cheng WH, Johnson LK. Superior inhibitory efficacy of butyrate over propionate and acetate against human colon cancer cell proliferation via cell cycle arrest and apoptosis: linking dietary fiber to cancer prevention. Nutr Res. 2020 Nov;83:63-72. doi: 10.1016/j.nutres.2020.08.009.
Zhang J, Yi M, Zha L, Chen S, Li Z, Li C, Gong M, Deng H, Chu X, Chen J, Zhang Z, Mao L, Sun S. Sodium Butyrate Induces Endoplasmic Reticulum Stress and Autophagy in Colorectal Cells: Implications for Apoptosis. PLoS One. 2016 Jan 19;11(1):e0147218. doi: 10.1371/journal.pone.0147218.
Zheng N, Gao Y, Zhu W, Meng D, Walker WA. Short chain fatty acids produced by colonizing intestinal commensal bacterial interaction with expressed breast milk are anti-inflammatory in human immature enterocytes. PLoS One. 2020 Feb 21;15(2):e0229283. doi: 10.1371/journal.pone.0229283.
Zou F, Qiu Y, Huang Y, Zou H, Cheng X, Niu Q, Luo A, Sun J. Effects of short-chain fatty acids in inhibiting HDAC and activating p38 MAPK are critical for promoting B10 cell generation and function. Cell Death Dis. 2021 Jun 7;12(6):582. doi: 10.1038/s41419-021-03880-9.